Knowing and wise, Jay is an integral part of the lab that technically works in another building. The balance is disturbed even when someone woke up on the wrong side of the bed.
Dianionic Bis(Phosphido) Pincer Ligands for High-Valent Early Transition
Synthesis, Solid-State, and Solution-State Behavior of a Palladium(II) Halide
Rapid double deprotonation of bis(phosphine)pyridine can be achieved quantitatively with KHMDS or benzylpotassium in THF to afford the dipotassium bound bis(phosphide) 12. Unlike 13, this bis(phosphide) is monomeric in the polyib unit , the steric charge of the tert-butyl substituents on the phosphorus atoms.
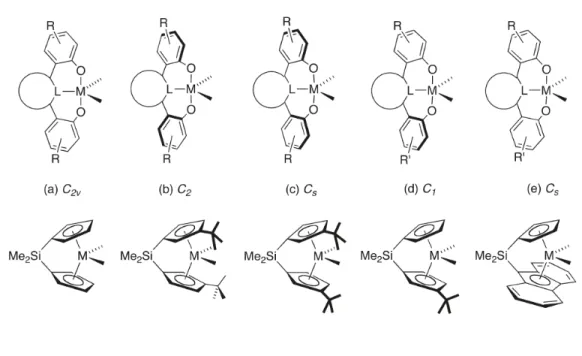
Ethylene Polymerization and Ethylene/1-Hexene Copolymerization
First introduced by our group in 1990 as scaffolds for scandium(III) catalysts,4 they have since been explored as ligands for Group 4 metals (Figure 3.1a).5 The absence of a second cyclopentadienyl ring also . When coordinated to furan- or thiophene-linked ligands, the titanium(IV) complexes oligomerize propylene with high activity (105 g mmol-1 h-1). Furthermore, the 13C NMR spectrum reveals only a singlet at δ 29.8 ppm corresponding to the methylene backbone of linear polyethylene (see Figure 3.3 for an example).
In the presence of different amounts of comonomer (1-hexene), the precatalysts 1-ZrBn2, 2-ZrBn2 and 3-VCl(THF)2 can be activated by MAO under ethylene (Table 3.2). Compared to the activity in the absence of comonomer, the activity is reduced by more than twofold with 80 mM (1 v%) 1-hexene. 13C NMR spectrum of an ethylene/1-hexene copolymer generated by the precatalyst 3-VCl(THF)2 in the presence of 160 mM 1-hexene.
13C NMR spectrum of ethylene/1-hexene copolymer generated by precatalyst 3-VCl(THF)2 in the presence of 320 mM 1-hexene.
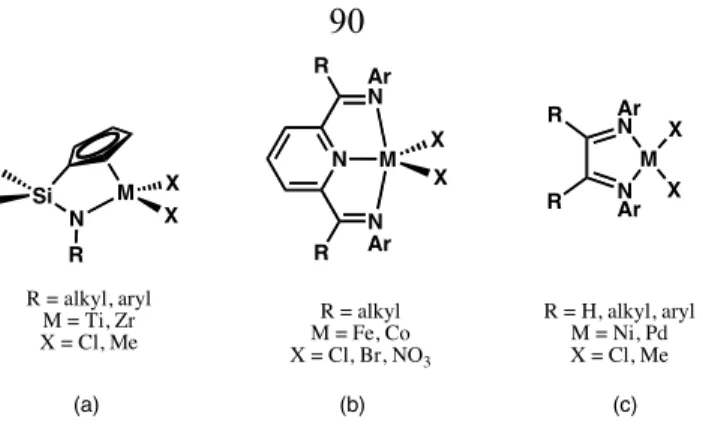
Activator-Free Olefin Oligomerization and Isomerization Reactions
Here we report an air- and water-tolerant bis(µ-hydroxy)palladium dimer supported by an α-diimine ligand that can be self-activated into an olefin oligomerization and isomerization catalyst. The nickel-based oligomerization step of the Shell higher olefins (SHOP) process represents one of the largest applications of homogeneous transition metal catalysis in industry. 1b Initiated by Keim, it is highly selective for the production of linear α-olefins in the C4-C30 range. Many nickel-based ethylene oligomerization catalysts supported by various bidentate monoanionic ligands have been reported since Keim's initial discovery.4 Almost all involve an active nickel hydride species.
Kinetic studies show that chain transfer is an associative process that requires the initial coordination of the monomer on the axial face of the tetracoordinate metal complex. 6a, 6b This has important implications in determining the overall behavior of the catalyst. It is thought that the orthoaryl substituents on the α-diimine ligands can block the axial approach of the monomer to the metal, inhibiting chain transfer relative to monomer insertion, which requires an olefinic approach to the in-plane vacancy. Indeed, complexes supported by α-diimine ligands with ortho isopropyl substituents (Ar = 2,6- C6H4(CHMe2)2) give polyethylene with a higher molecular weight than those supported by ligands with less bulky ortho methyl groups (Ar = 2 ,6 -C6H4(CH3)2).
Furthermore, nickel(II) complexes supported by para-only ligands exhibit ethylene oligomerization and propylene dimerization activity with a TON ~ 105 (Figure 4.1).7.
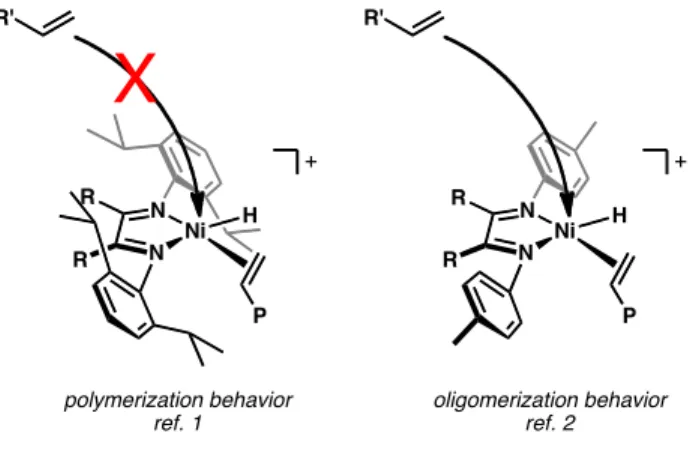
Formation of inert platinum(II) bis( µ -hydroxy) dimers observed by
For example, Vedernikov and co-workers12 reported that the reaction of 6 with O2 affords the bis(µ-hydroxy) dimer 7 via dependent sulfonate-assisted oxidation of the platinum(IV) and C–O bond coupling (Scheme 4.3). It should be noted that dissociation of 7 was not observed even under ethylene at elevated temperatures to regenerate 6. On the other hand, Sodeoka and co-workers13 reported that the dimer [(R-BINAP)Pd(OH)]22+ (8) is not chemically inert, but instead reacts with silyl enol ethers to cleave to 9, which catalyzes asymmetric Mannich reactions with imine substrates.
Mechanism of palladium(II) bis( µ -hydroxy) dimer-catalyzed Mannich
Mechanism of palladium(II) bis( µ -hydroxy) dimer-catalyzed alcohol
Aryl C-H bonds can also be activated, provided the metal is directed to the reacting C-H bond, ultimately producing a polydentate ligand. With allyl benzene, the pendant olefin coordinates to the metal in the activated C-H product, while the nitrogen coordinates to generate a cyclometalate in the reaction with aryl oximes. This suggests that the reaction is not adversely affected by water released after coordination and activation of the organic substrate.
Stoichiometric C-H activation behavior of platinum(II) complexes 11a and
Mechanism of C-H activation of by platinum(II) bis( µ -hydroxy)
Stoichiometric C-H activation behavior of palladium(II) bis(µ-hydroxy)
A half-order dependence of 4a and a first-order dependence of the indene and co-solvent concentrations suggest a mechanism involving solvent-assisted dissociation of the dimer to monomeric solvent adduct 14 followed by rate-limiting displacement of solvent by indene to generate substrate. bound intermediate 15, which then undergoes C-H activation. It is expected that the palladium(II) and platinum(II) monomers react with indene via the same mechanism.
Mechanism of C-H activation of by palladium(II) bis(µ-hydroxy) dimer
Furthermore, the reaction of 4a (t1/2 ~ 2 days) is significantly accelerated in the presence of a small amount of nucleophilic co-solvent (t1/2 ~ 5 minutes), such as trifluoroethanol (TFE) or MeOH. PtCl62- and Br2 resulted only in the release of halogenated (oxidized) organics and unreactive bis(µ−halo) metal dimers. The reasons for the different activation mechanisms of palladium(II) and platinum(II) dimers are unclear.
However, the formation of an active species from palladium(II) dimer 4a is simply accelerated in the presence of nucleophilic co-solvent and encouraged us to investigate further dimer reactivity, namely the stoichiometric C-H bond activation of linear olefins and unactivated alkanes under mild conditions. The original reported synthesis16 of palladium(II) dimer 1a from bis(aquo) monomer 2a is problematic and unreliable. Silver-assisted halide abstraction in THF yields bis(solvento)complex 18a, which when treated with water in the presence of a polymer-supported base (“polymer-bound diethylamine”) yields dimer 4a in 70% overall yield from 17a , which is a diagnostic, upfield singlet in the 1 H NMR spectrum corresponding to the hydroxy bridge protons (Figure 4.3).
In the presence of deuterated alcohol, these signals disappear within several hours due to H/D exchange.
Synthesis of palladium bis(µ-hydroxy) dimers 4a and 4b
As expected, reaction of any other linear hexene isomer with precatalyst 4a gives an equivalent mixture of hexene isomers. The isomerization/oligomerization catalyst obtained from 4a appears to be quite insensitive to the presence of water or air, as the reaction rate and isomer/oligomer distribution remain essentially unchanged upon addition of 2000 equivalents of H2O/Pd (Figure 4.8), or operating under 1 atm pure O2 (Figure 4.9). Indeed, rigorous exclusion of water using dried, distilled solvents and molecular sieves does not appear to affect the reaction rate or isomer/oligomer distribution (Figure 4.10).
However, addition of the olefin substrate at 40 °C results in significant precipitation of black Pd, suggesting that one or more post-activation species are temperature sensitive (Figure 4.11). Precatalyst 4a oligomerizes propylene with a TOF of 600/h to provide a Schulz–Flory distribution that can be measured by GC and fractional distillation (Figure 4.14). Reaction of ethylene (1 atmosphere) with 0.1 mol% dimer 4a under reaction conditions gives a mixture of C4–C18 branched oligomers (eq 10).
Precatalyst 4a oligomers ethylene with a TOF of 600/h to provide a Schulz-Flory oligomer distribution that can be quantified by GC and fractional distillation (Figure 4.15).
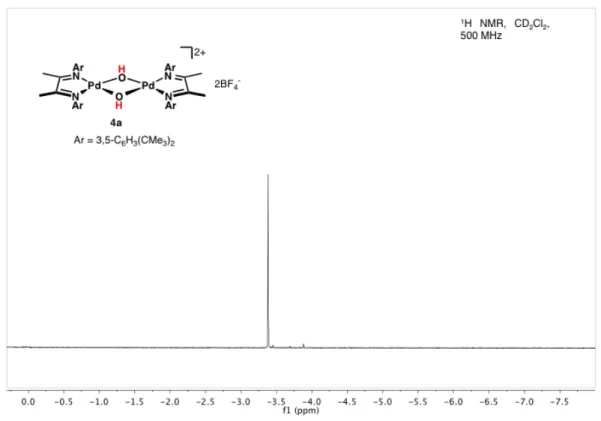
Plausible mechanism of olefin isomerization/oligomerization by 4a
Halide abstraction of 17a generates bis(µ−chloro)dimer 21 in good yield, which can be increased to 22 after treatment with allyltributylstannane. Indeed, a small amount of linear hexadiene isomers can be detected by GC when 1-hexene is treated with precatalyst 4a . Under an ethylene atmosphere, acetaldehyde can be detected and quantified by VT-NMR using an internal standard (Figure 4.19).
With 1-hexene or ethylene, when 0.1 mol % of dimer 4a is used, about 0.2 mol % of the oxidized product is detected; using 1 mol% of 4a yields 2 mol% of the oxidized product. That the total amount of oxidized products is approximately the same as the original amount of palladium(II) means that the activation of 4a by reaction with the olefin involves two different pathways: a C-H bond activation/dehydrogenation sequence and a Wacker-like palladium(II)-mediated oxidation (Scheme 4.13). Selected region of the 1H NMR spectrum showing the presence of catalytic amounts of 2-hexanone after exposure of 1-hexene to 0.1 mol% 4a under reaction conditions for 1 h.
Selected region of 1H NMR spectrum indicating the presence of catalytic amounts of acetaldehyde after exposure of ethylene (1 atm) to 0.1 mol% 4a under reaction conditions for 1 h.
 (22)](https://thumb-ap.123doks.com/thumbv2/123dok/10402327.0/168.918.308.647.111.422/figure-isotropic-non-refined-ball-stick-depiction-budab.webp)
Mechanisms of activation of dimer 4a
The reaction of 4a with ethylene, propylene or 1-hexene in the absence of TFE starts on the order of days. We prepared a bis(µ−hydroxy) dimer with such substituents, [(iPrDABMe)Pd(OH)]2(BF4)2 (4b), in the hope of developing an activator-free polymerization catalyst capable of is to self-activate in the presence of olefin substrate. Instead, halide abstraction using a smaller cosolvent such as MeCN gives bis(acetonitrile) adduct [(iPrDABMe)Pd(MeCN)2][BF4]2 (18b) in high yield, which leads to 4b taken under basic conditions in the presence of water.
Because an active catalyst is never accessed in the first place, 4b (unlike 4a) can be re-isolated after heating to 100 °C for 3 days in the presence of olefin and nucleophilic co-solvent. The reaction was filtered through Celite and concentrated in vacuo to afford 21 (101 mg, 0.159 mmol) as a bright orange powder. The solution was concentrated in vacuo to give a yellow powder, which was dissolved in CH2Cl2 and filtered through Celite to remove residual AgCl.
Using the gas bulb, sufficient air was added to the flask to ensure that the pressure in the vessel reached 1 atm when warmed to room temperature.
 (24) at the 50% probability level](https://thumb-ap.123doks.com/thumbv2/123dok/10402327.0/174.918.284.651.128.611/figure-thermal-ellipsoid-representation-dab-och-probability-level.webp)
Synthesis of bis(enolate) bridged complex 25
In support of a mechanism involving a catalytically active palladium(II) hydride (3), we prepared the unreported tBuDABMe supported palladium(II) methyl cation (2a) by the Brookhart method (COD scheme) PdMe2 (COD = 1,5-cyclooctadiene) was treated with to the tBuDABMe ligand in Et2O to provide the dimethyl precursor 1a in good yield.
Synthesis of [( tBu DAB Me )Pd(Me)(OEt 2 )](BAr 4 ) (2a)
The 1HNMR spectrum of dimer 4a, including the signal corresponding to the hydroxyl bridging protons, is completely unchanged in pure TFE in the absence of olefins, even at elevated temperatures (Figures 4.22). This is perhaps not surprising, given that C−H activation of the indene with 4a, a process that also requires dimer dissociation, takes 2 days in the absence of a nucleophilic cosolvent.16 These observations suggest that the first steps of activation, including dissociation of the dimer, appears to require TFE and the olefin. Indeed, 4a is stable at 40–60 °C in the presence of TFE or olefin, but upon heating in the presence of TFE and olefin, the dimer decomposes to palladium metal within 10 min.
In addition to aiding in catalyst activation, TFE appears to stabilize one or more intermediates in the catalytic cycle. In the absence of TFE and olefin, the palladium(II) methyl cation 2a is relatively stable, but when the olefin is added, significant decomposition to palladium metal is observed after one hour. The reaction was stirred for 3 h at room temperature, then filtered through celite and concentrated in vacuo to give 18a as a light orange powder (18.8 g, 21.3 mmol).
CH 2 Cl 2 (17 mL) and TFE (3 mL) were added via syringe to give a yellow solution which was degassed by two freeze-pump-thaw cycles.
![Figure 4.21. Isomerization (top) and oligomerization (bottom) of 1-hexene. Reaction conditions: 1-hexene (6.40 mmol), [( tBu DAB Me )Pd(Me)(OEt 2 )][BAr’ 4 ] (2a, Ar’ = 3,5-C 6 H 3 (CF 3 ) 2 ) (0.2 mol%), CH 2 Cl 2 (8 mL), TFE (4 mL), a](https://thumb-ap.123doks.com/thumbv2/123dok/10402327.0/177.918.172.751.113.822/figure-isomerization-oligomerization-hexene-reaction-conditions-hexene-mmol.webp)