To date, probabilistic formulations have mostly involved kinematic descriptions of particle motion and transport with limited elucidation of the associated mechanics. This work highlights the need for: (1) a conceptualization of how particles interact energetically with the surface over which they travel, (2) a demonstration of how particle angularity and size affect downward and lateral transport distances, and (3) an understanding of the mechanical basis of particle deposition. Builder from Furbish et al. 2020a), my focus on particle energy associated with particle–surface collisions allows me to elucidate elements of the mechanics involved in sediment detachment, hence the distribution of particle travel distances on hillslopes relative to the sediment flux.
The clickety-clack sounds emitted by the particles as they move down the slope is a sonic manifestation of the irreversible energy loss that occurs (Furbish et al., 2020b). In this situation, probabilistic formulations of sediment transport begin with the one-dimensional association form of the Exner equation (Tsujimoto, 1978; Parker et al., 2000). E(x0,t)fr(x−x0;x0,t)dx0, (3.1) where E(x,t) is the volume rate of withdrawal at position xand times, and fr(r;x,t) is the dis- distribution of travel distances r of particles whose motions start at positionx.
The spatial velocity of particle deposition in the interval is dN/dx and depends on the energy states of the particle in the cohort. The arithmetic average of the energy for a large number of particles N is Ea=hEpi, where the square brackets indicate the average of the ensemble of energy states of the particles. Using the full formulation developed in Furbish et al. 2020a), the conservation of the total energy of the cohort is given by.
LargeKleads for net particle heating represented by a heavy-tailed form of the Pareto distribution.
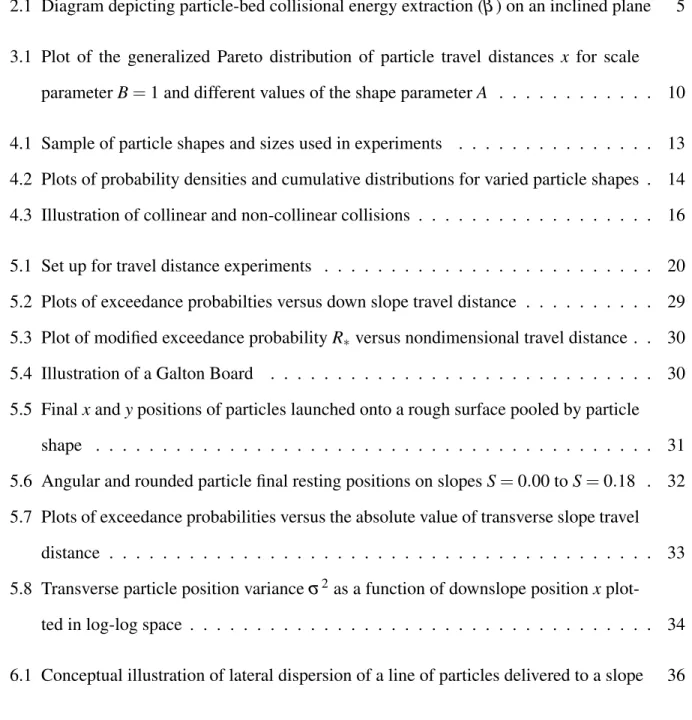
Experiments
However, the change in translational energy ∆Ep is partitioned between deformational friction, rotational energy and transverse motion, so the coefficient εx (and thus the factor βx) cannot simply represent a coefficient of restitution - although particle collision theory suggests that this coefficient represents effects of normal and tangential coefficients of restitution as normally defined (Brach, 1991; Stronge, 2000). Glass marbles, of similar size to the rounded and angular particle groups, are not shown.
Results
In comparison, the probability distributions for energy extraction with collision for both rounded and angular natural particles have greater variance on the smooth surface (Figure 4.2b) than on the rough surface (Figure 4.2d). Probability distributions for natural rounded and natural angular particles plot close to each other and show much less variation in energy extracted by collision on the rough surface compared to . The shift inβz with particle category is interpreted to be a result of the probability of collinearity.
Under frictionless conditions, the spin of the sphere would be negligible and the rebound would remain aligned with the center of mass and the point of contact (Lim and Strong, 1994). If the rolling sphere or surface is roughened, the center of mass remains in line with the contact point, but the rebound will not be collinear since the rotation of the sphere produces a finite friction torque that results in a change in the rebound path. Assume that the surface of the cube and the surface on which it is thrown are frictionless.
Only the points of the cube are locations of non-trivial probability for a collinear collision. Surface roughness further reduces the probability of collinear collision and affects the torque on the particle, leading to an increased conversion of translational energy into rotational energy during surface collision. The shape of the particle together with the surface characteristics and the incident motion conditions determine the repulsive motion.
In contrast, for a non-spherical (angular or rounded) particle with the same angle of incidence, a large number of outcomes are possible depending on the orientation of the particle, each outcome involving a different extraction of incident kinetic energy. The files “Rounded colinear.avi” and “Angular colinear.avi” provided in the supplementary materials show examples of near-collinear collisions on the solid plate with minimal roughness. These examples are more characteristic of the general behavior of the particles during these experiments, especially on the rough surface.
Marbles on the smooth surface experience the least energy extraction of the three shape groups, and angular particles experience slightly more energy distribution than naturally rounded particles. On the rough surface with effectively no chance of collinear collision, energy is extracted at a much higher rate for all dropped particles. Although marble behavior is more similar to natural particles on the roughened surface, the sphere is generally not representative of effects of shape for natural particles with different angularity.
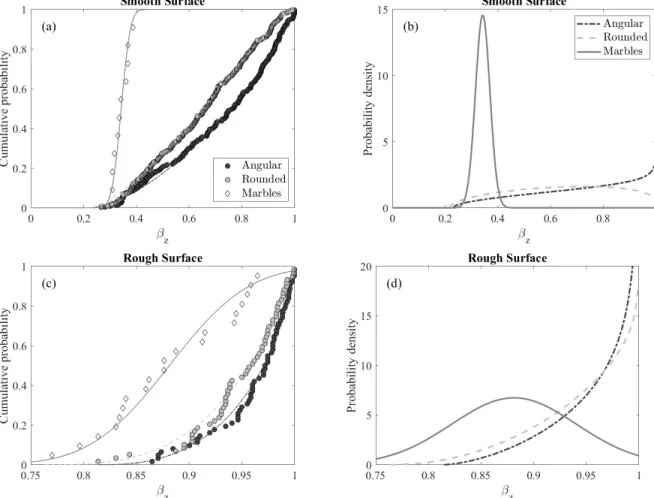
Experiments
Downslope travel distance
A gravel-sized particle is placed on the low-friction (glossy cardboard) cradle surface at the base of the 8-inch pendulum arms. The bend in the initial exceedance probability plots (not shown) reflects the uniformity of the launch velocities followed by a finite distance over which randomization of the movements occurs. The deflection does not necessarily correspond directly to flight distance, and the details of the physics prior to randomization are unclear (Furbish et al., 2020b).
Modified experimental travel distance data are fitted to the generalized Pareto distribution and plotted using exceedance probability plots (Figure 5.2). In addition, quartile-quartile plots (not shown) were used by Furbish et al. 2020b) to assess consistency with general Pareto distribution fits. Due to the small sample size, overfitting of tails in semi-log plots was avoided (see Appendix A in Furbish et al.
The fits and parameter values shown in Figure 5.2 and Table 5.1 are specific cases of the generalized Pareto distribution. The deviations from the distribution tails are highlighted using this plotting method and the censored data associated with slopes above 0.18, although used during the calculation, are not included in the plot. High-speed imaging of initial particle impacts on the surface reveal a variety of impact geometries that appear to influence motions during subsequent particle displacement (Supplementary Materials, Vanderbilt University Institutional Repository, http://hdl.handle.net/1803/ 9742).
After initial contact with the surface, downward movements appear to transition to jumping and rotating movements, as shown in the files "Angular 18%slope.avi" and "Angular 28%slope.avi". Downward travel distances become longer for both angular and rounded particles due to decreasing efficiency of frictional cooling (Ki >1) with increasing slope, partly due to less frequent particle collision with the surface and increased conversion of translational energy to rotational energy (Figure 5.2) ). For the same slopes, angular particles are removed on the surface, although a significant number of particles reached the wood bumper at the bottom of the concrete slope.
These rejected movements are included in the calculations of the exceedance probabilities and contribute to the heavy nature of the generalized Pareto distributions on these slopes. The small particles exhibit a behavior intermediate between that of the larger angular and round particles, which is better described by two or more distributions. This is consistent with the idea that mass does not appear in Kinor's formulation, but does appear in Eq.
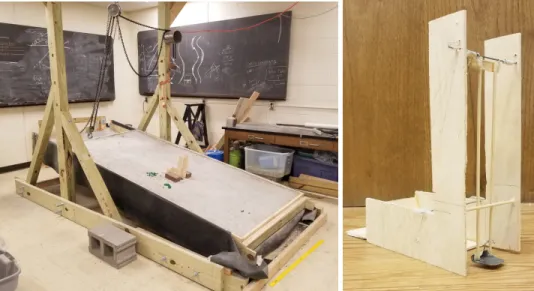
Lateral spreading
This quasi-random walk of the particles on a Galton plate results in a binomial distribution of net transverse displacements, which transitions to a Gaussian distribution with increasing number of pin rows. Mechanical spreading only works in the presence of advection due to forcing forces such as fluid drag or, as in the case of the Galton plate, the effect of gravity. In the case of the Galton plate, the motion of the particle is affected in a bottom-up manner by the roughness elements on the surface.
This surface roughness is only part of the problem with particle motion on hillslopes, where particle characteristics—particularly angularity—provide a top-down influence on the randomization of motion. Final resting positions show that, in addition to spreading down slopes with travel distances consistent with shapes of the generalized Pareto distribution, particles spread laterally along the surface perpendicular to the mean downward motion (Figure 5.5). The average lateral position is shown in red and does not vary significantly from the center line of the slope=0.
Marked transverse scattering is observed not only in the farthest particles, but in the overall population compared to angular particles at the same slope. By visualizing the transverse travel distance for angular and rounded particles using exceedance probability plots, I am able to observe trends in energy extraction with increasing slope in the same way as before with downward travel distance. The deviations for small values of downhill travel distancex should be viewed with skepticism due to the small sample size used in the calculations.
On a hill slope, there is a fixed amount of potential energy that can be dissipated before the particle reaches the bottom of the slope. Particles have maximum potential energy available to be converted to kinetic energy at the crest of the hill slope and have a maximum kinetic energy at some point between this initial position and deposition site. Tails of the data plotted are not included in the fit as these variance values are distorted by low sample numbers, at small downslope positions, or truncation of data, at larger downslope positions.
Angularity, in addition to surface roughness, introduces randomness of motion and is thus an important element of the transport problem. Lateral particle dispersion is a key component of transport in many environments, and a full description of sediment transport requires a better understanding of the observed kinematics and underlying mechanics. For completeness with respect to future work, I provide here the two-dimensional versions of the particle flux induction shapes and the Exner equation.
Only the one-dimensional version of the flux coupling form has been presented previously ( Furbish et al., 2012b ; Furbish and Roering, 2013 ; Furbish et al., 2017 ). E(x0,y0)fr,s(x−x0,y−y0;x0,y0)dx0dy0 (A.7) kucb is the volume concentration of sediment surface particles and E(x,y) is the volume compaction rate.
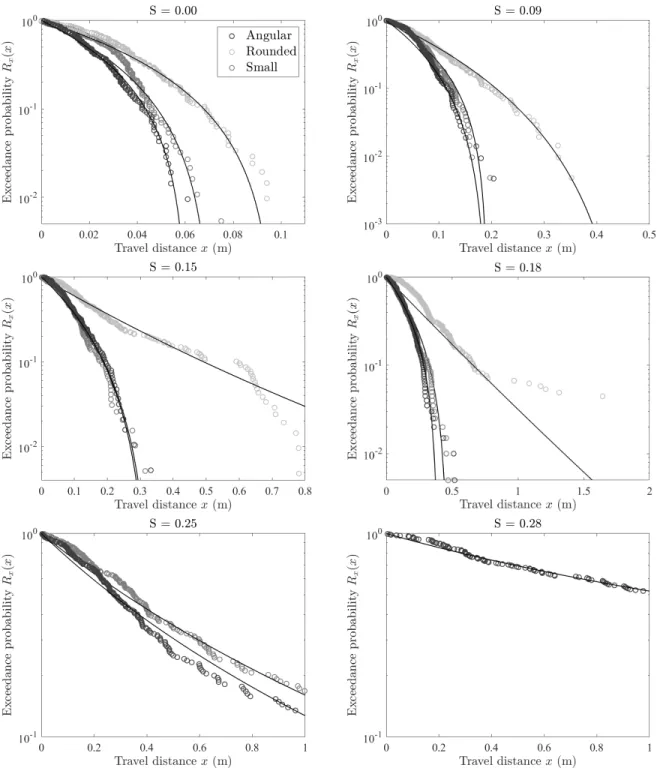