INTRODUCTION
Background / Generalities
Chromium (Cr) is a transition metal of great industrial importance, but poses a great threat to the environment. More recent researchers have found that some plants can effectively reduce the mobility and reactivity of Cr(VI) in the environment.
Chromium
The decrease in protein content in Cr(VI)-exposed plants suggests that proteins are easily susceptible to oxidative stress damage induced by Cr(VI) in these plants. The significant Cr(VI) removal efficiency and rate of Cr(VI) removal achieved in T.
Treatment of chromium contaminated wastewater
Problem statement
Most developing countries have warm tropical and subtropical climates conducive to high biological activity and productivity, and are therefore most suitable for wetland treatment systems (Zhang et al., 2015). These multiple pollutants can be in the same form, e.g. soil contamination with different types of heavy metals, or can be in different forms, e.g.
Aims and objectives
Therefore, this research work is an effort towards addressing the environmental pollution due to the discharge of industrial effluent containing Cr(VI) and its mitigation using an indigenous plant species. Detailed studies on chromium uptake, tolerance and distribution by plant species along with their function and application in a constructed wetland system were conducted to fulfill the purpose of this thesis.
Organization of the thesis
Although some variation was observed in the percentage removal of Cr(VI) and the co-ions in each of the experimental runs, the removal was generally slow and steady for Cr(VI) and the co-ions. The increase in the Cr(VI) sorption equilibrium constant value with temperature reveals an increase in the heat of sorption with an increase in temperature, which clearly shows that Cr(VI) sorption further to T.
LITERATURE REVIEW
Chromium
- Physico-chemical properties of chromium
- Uses of chromium
- Sources and concentration of chromium in the environment
- Health effects of chromium
In contrast, Cr(VI) is considered the most harmful form of Cr with a strong oxidizing potential. Wastewater from these tanneries is loaded with about 40% Cr, which is used in the form of Cr(VI) and Cr(III) salts (Sundaramoorthy et al., 2010).
Chromium removal methods
- Physico-chemical methods of chromium removal
- Biological methods of chromium removal
In plants and many other organisms, reducing agents, such as NAD(P)H, FADH2, some pentoses, and glutathione in the cell pool, reduce Cr(VI) to Cr(III) (Hossain et al., 2012). During this conversion, the transient formation of unstable Cr states occurs leading to the formation of free radicals, which induce oxidative stress conditions in plants (Sharma et al., 2012).
Phytoremediation overview
- Removal of chromium by phytoremediation
- Plants with potential of chromium phytoremediation
In contrast, Henriques et al. 2010) reported pigment damage due to Cr(VI) by performing a photochemical experiment with irradiated chloroplasts. Similar results were obtained in celery seedlings grown in the presence of Cr(III), where Cr was accumulated mainly in the roots (Scoccianti et al., 2006).
Chromium tolerance and detoxification mechanisms in plants
- Tolerance mechanisms
- Detoxification mechanisms
Lahouti et al., (2008) reported the accumulation of Cr as inclusion bodies in the cell wall of root cortical cells in Raphanus sativus. The high amount of Cr accumulated mainly in the cell walls and vacuoles of the fourth or fifth cortical layer.
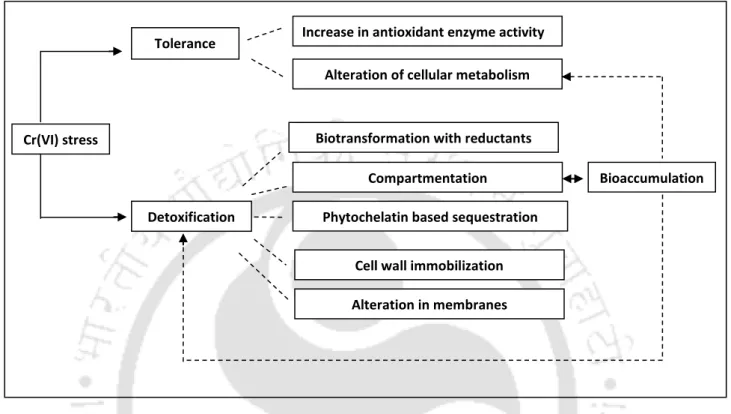
Chromium transport and uptake mechanism in plants
In contrast, no differences in the uptake of Cr(III) or Cr(VI) were observed in Phaseolus vulgari (Nath et al., 2009) and Triticum aestivum L. vermicompos increases the Cr bioavailability and thus increases the uptake of Cr by plants (Jean et al., 2008). This is determined by the fact that chelating agents possess functional groups capable of Cr absorption and conversion.
Replenishment of contaminated soil with vermicompost has been reported to further enhance plant biomass growth, thereby favoring the bioaccumulation potential of plants as found in Sorghum (Revathi et al., 2011) and Helianthus annus (Jadia and Fulekar, 2009).
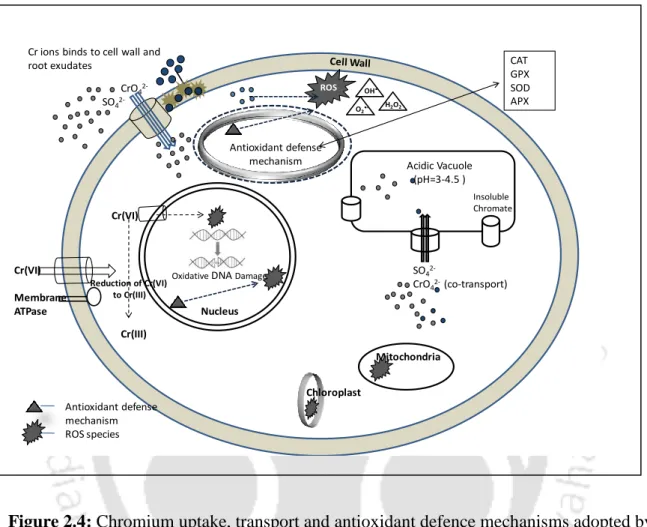
Chromium removal in constructed wetland systems
- Types of constructed wetlands
- Disposal and reuse of chromium accumulated plant biomass….… 50
The most common Cr accumulator plant species used in CWs for Cr removal are the Phragmites australis, Typha latifolia (Vymazal and Brezinova, 2016), Leersia hexandra (Liu et al., 2015), Brassica juncea (Bluskov et al., 2005 ) and Dicoma niccolifera (Banach et al., 2012). The process involves a passive uptake mechanism and mainly depends on the "affinity" between a biosorbent and sorbate which can result from complex formation, metal chelation, ion exchange, adsorption and microprecipitation (Sud et al., 2008). The performance of an adsorbent depends on many factors, such as pH, the ionic form of the metal to be removed, and competition from other organic or inorganic ions for available binding sites on the adsorbent (Anwar et al., 2009).
Different types of biomass have been investigated for Cr(VI) removal, which include agricultural waste, plant biomass and industrial by-products (Pakshirajan et al., 2013). A pilot-scale reactor study reported 98.5% metal recovery (Ni, Zn, Cu, Co or Cr) in the char formed by pyrolysis and gasification of hyperaccumulating plant biomass (Koppolu et al., 2003). Controlled combustion, where ash with a high metal content is recovered, is another option for disposal of such plant biomass (Revathi et al., 2011).
In addition, volume reduction processes, such as composting and compaction of Cr-accumulated biomass, have been proposed as post-harvest biomass treatment (Shukla et al., 2009).
Chemicals and reagents
Collection and maintenance of plant species
Chromium (VI) tolerance, accumulation and localization
- Screening of different plants species
- Chromium accumulation in T. pallida
- Extraction and estimation of total chromium
- Separation and determination of chromium in different subcellular
- Plant growth in presence of chromium
- Separation of the subcellular fractions
- Energy dispersive X-ray analyses
54 plant species were grown under hydroponic conditions and maintained under conditions as previously described in section 3.2. Translocation factor (TF) was expressed as the ratio of Cr(VI) concentration in the plant shoot and root biomass and was calculated according to Eq. For estimation of total Cr content, plant parts were harvested after 30 and 60 days of Cr treatment, rinsed thoroughly with distilled water to remove any adsorbed metal on the root surface prior to digestion and analyzes as further described.
The solutions were changed every 7 days to maintain the constant Cr(VI) concentration during the 30 day experimental period. The plants were harvested after 30 days of treatment and the leaves, shoots and roots were separated. HNO3, total Cr concentration were measured by atomic absorption spectrophotometry as previously mentioned in section 3.3.2.1.
Schematic representation of steps followed in separation of organelles sedimented by differential centrifugation in presented in Fig.
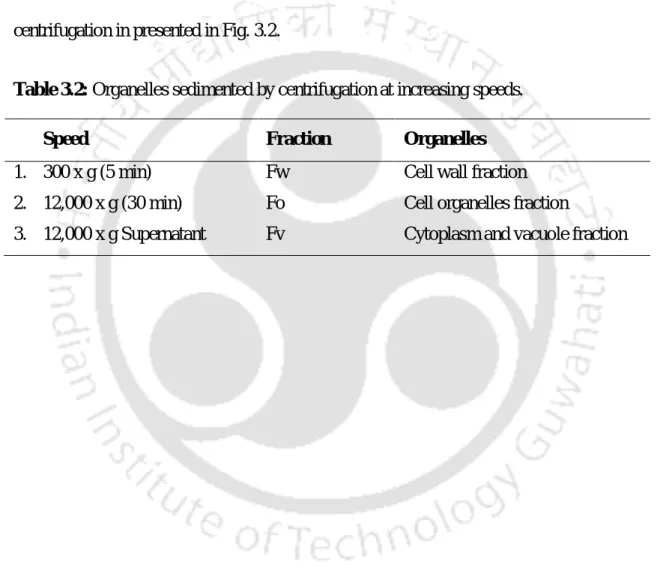
Antioxidant enzyme and biochemical changes in T. pallida due to chromium
- Estimation of lipid peroxidase activity
- Estimation of catalase activity
- Estimation of peroxidase activity
- Estimation of ascorbate peroxidase activity
- Extraction and estimation of proline content
- Estimation of chlorophyll content
- Estimation of total carbohydrate content
- Estimation of total protein content
The reaction mixture was then added to 0.5 ml of enzyme extract and enzyme activity was measured by monitoring the decrease in absorbance at 240 nm due to H 2 O 2 consumption. Peroxidase activity was determined based on the oxidation of guaiacol, which was measured by an increase in absorbance at 470 nm (Ambreen et al., 2000). 4 mL of toluene was added to the mixture and mixed vigorously using a test tube stirrer for 20 seconds, the toluene phase containing the chromophore was separated from the aqueous phase using a separatory funnel, and the absorbance was measured at 520 nm in a spectrophotometer using a reagent blank.
About 100 mg of plant tissue sample was hydrolyzed for 3 h in a water bath with 5 ml of 2.5N HCl. 63 For the Anthrone method, 2.5 ml of anthrone reagent was mixed with 0.5 ml of an appropriately diluted sample taken in a series of test tubes and heated in a boiling water bath for 15 minutes. A suitable blank is prepared by mixing 2.5 ml of Anthrone reagent with 0.5 ml of Milli Q water and heating in a boiling water bath for 15 min.
2 ml of alkaline copper sulphate reagent was mixed with 0.5 ml of a suitably diluted sample taken in a series of test tubes.
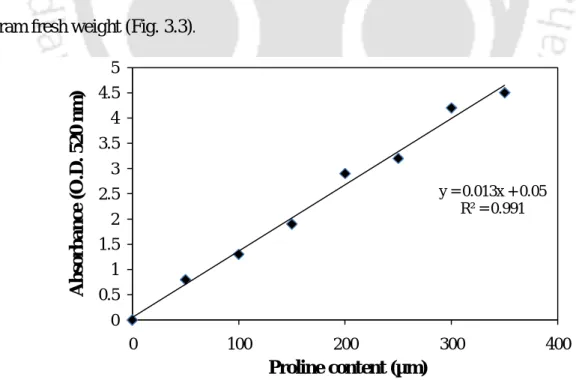
Effect of co-ions on chromium (VI) uptake by T. pallida
- Plackett–Burman (PB) Design of Experiments
- Chromium (VI) removal kinetics
- Estimation of sulphate
- Estimation of nitrate
- Estimation of phosphate
The intensity of the developed color was measured at 660 nm with a UV-visible spectrophotometer (Cary 100, Varian, Australia). The response in each of the experimental sets was expressed as % removal of Cr(VI) and coions and calculated using the following equation. The statistical software package MinitabTM (version 16, PA, USA) was used to analyze the experimental data.
Water samples were first filtered through filter paper (Whatman No. 1) and 50 ml of the filtrate obtained was mixed with 20 ml of buffer solution in a 100 ml volumetric flask. Total nitrate content was measured according to the standard salicylic acid method (Cataldo et al. 1975). The intensity of the color developed was measured at 410 nm using a UV-visible spectrophotometer (Cary 100, Varian, Australia).
The intensity of the developed color was measured at 880 nm with a UV-visible spectrophotometer (Cary 100, Varian, Australia).
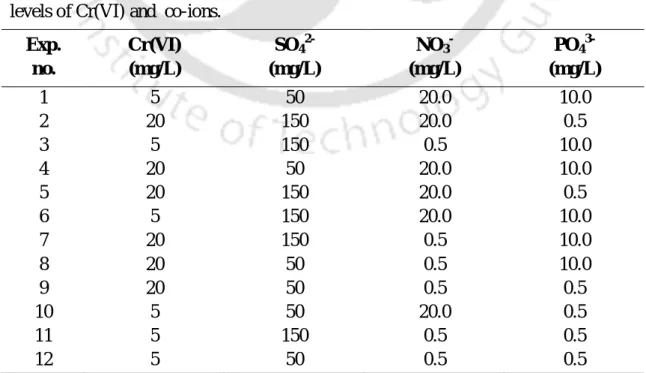
Continuous chromium (VI) removal from wastewater by T. pallida using
- Constructed wetland design
- Continuous chromium (VI) removal experiments
- T. pallida growth and chromium bioremoval
- Extraction and estimation of chromium (VI)
- Extraction and estimation of total chromium and chromium (III) 77
- Preparation of T. pallida plant biomass
- T. pallida biomass characterization for chromium (VI) biosorption. 78
- Influence of chromium (VI) biosorption process parameters
- Chromium (VI) sorption kinetics and isotherm
- Chromium (VI) sorption thermodynamics
In the present work, tolerance index (TI) of different plant species was determined for screening and selection of Cr(VI) accumulating plant species. It was found that 63.2% of the total Cr was present in the root cell wall fraction (Fw), while 28% Cr was present in the soluble fraction (Fv). Negative correlation between initial Cr(VI) concentration and protein content could be observed in the absence of the co-ions.
Thus, in the implanted system, Cr(VI) removal rate was higher than the control system when operated at the same Cr(VI) loading rate. In the pH 4 CW unit, the primary Cr(VI) removal mechanism is attributed to the adsorption of Cr(VI) ions. Therefore, Cr was mainly retained in the bed of the wetland media (soil and sand).
The results show that the percentage of Cr(VI) removal efficiency increased with an increase in biosorbent dose.
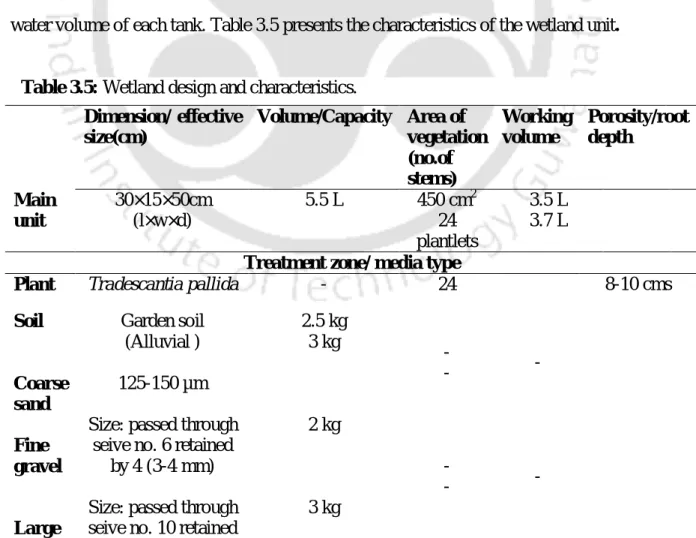
RESULTS AND DISCUSSIONS
Chromium (VI) tolerance, accumulation and localization by
- Chromium (VI) sorption isotherm parameters
- Thermodynamics of chromium (VI) biosorption onto T. pallida
The time profile of Cr(VI), SO42, NO3, PO43 removal in the different experimental runs is shown in Fig. Lipid peroxidation increased with a high initial Cr(VI) concentration in the absence of co-ions. The protein content was also low in control (A) and control (B) plant treated with 5 and 20 mg/L Cr(VI), respectively, in the absence of the co-ions (Fig. 4.14b).
The effect of metal loading level and influent pH was studied on Cr(VI) removal in CW units. A further increase in the Cr(VI) loading rate (20 mg/L/day) greatly reduced the Cr(VI) removal efficiency to 88% in the case of planted CW and to 77.8% in the case of the control system. In addition, this investigation revealed the importance of influent pH controlling the Cr removal mechanism in CW.
Thus, in this study, lower pH favored the biosorption of Cr(VI) in plant biomass than higher pH (Moussavi and Barikbin, 2010). The distribution of Cr in the CW indicated that the soil acts as a major Cr sink, sequestering up to the total Cr supplied to the system. Phytoremediation of Cr (III) by Ipomonea aquatica (water spinach) from water in the presence of EDTA and chloride, Effects of Cr speciation.
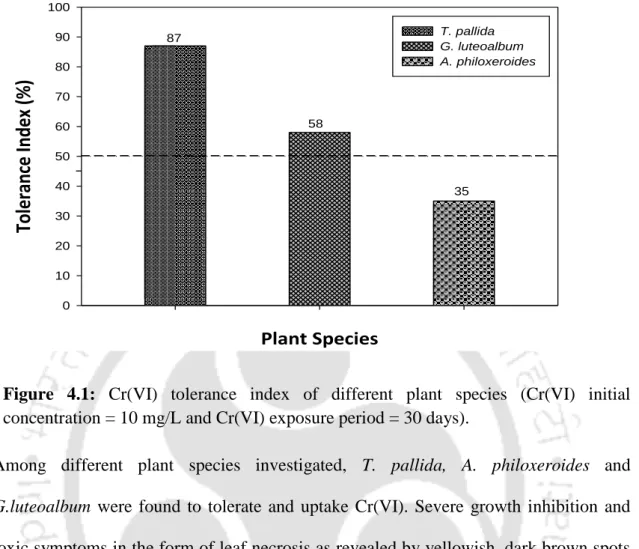
SUMMARY AND CONCLUSIONS
BIBLIOGRAPHY
LIST OF PUBLICATIONS