The second is my use of the imaginary numerito to impose the metric in special relativity. At the top of the list, I gratefully acknowledge the contributions of Wayne Saslow (Texas A&M University) and Glenn Smith (Georgia Tech).
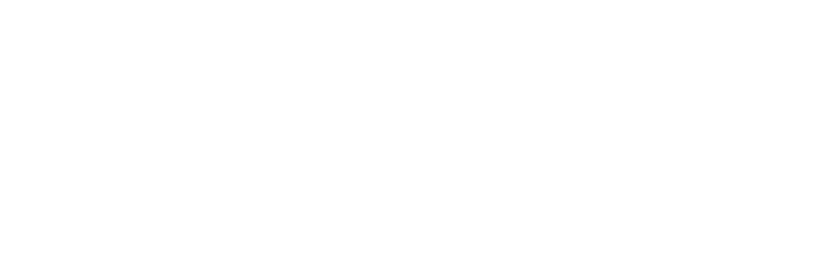
Introduction
Vectors
- Cartesian Coordinates
- Cylindrical Coordinates
- Spherical Coordinates
- The Einstein Summation Convention
- The Kronecker and Levi-Civit`a Symbols
- Vector Identities in Cartesian Components
- Vector Identities in Curvilinear Components
The volume element in spherical coordinates isd3r=r2sinθdrdθdφ. The unit vectors are related by. The identity on the left-hand side of (1.37) and the invariance of the Levi-Civit`a symbol with respect to cyclic permutations of its indices gives.
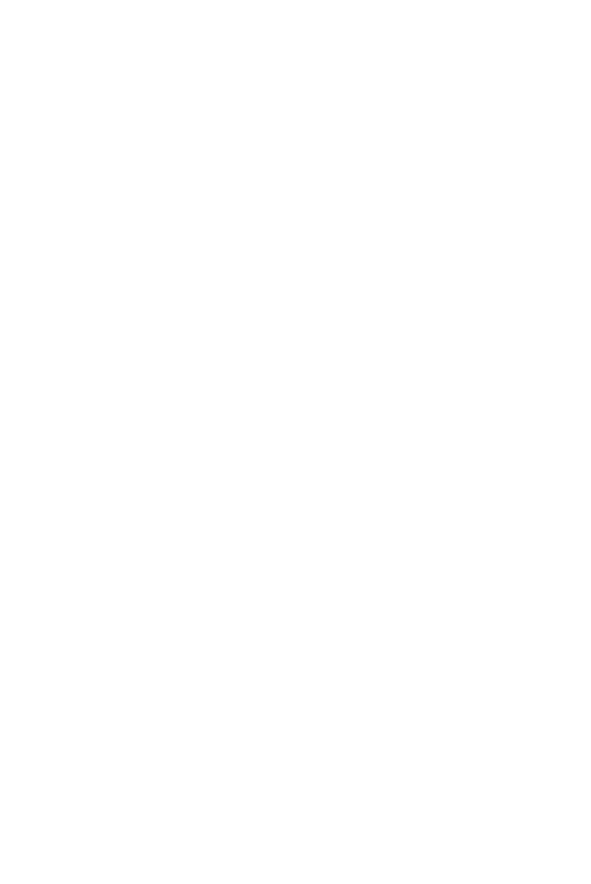
Two Identities for ∇ × L
- Derivatives
- Functions of r and | r |
- Functions of r − r
- The Convective Derivative
- Taylor’s Theorem
- Integrals
- Jacobian Determinant
- The Divergence Theorem
- Green’s Identities
- Stokes’ Theorem
- The Time Derivative of a Flux Integral
- Generalized Functions
- The Delta Function in One Dimension
- The Principal Value Integral and Plemelj Formula
- The Step Function and Sign Function
- The Delta Function in Three Dimensions
- Some Useful Delta Function Identities
- Fourier Analysis
- Parseval’s Theorem
- The Convolution Theorem
- A Time-Averaging Theorem
- Orthogonal Transformations
- Passive Point of View
- Active Point of View
- Cartesian Tensors
- Reflection, Inversion and Pseudotensors
Our treatment of Faraday's law uses the time derivative of the surface integral, where the surface S(t) moves because its individual surface elements move with velocityυ(r, t). In curvilinear coordinates, this is indicated by the restriction on the right-hand side of (1.113) and the shape of the volume elements for cylindrical and spherical coordinates.
![Figure 1.3: A surface S(t) [bounded by the solid curve labeled C(t)] changes to the surface S(t + δt) (dashed curve) because each element of surface moves by an amount υ δt.](https://thumb-ap.123doks.com/thumbv2/azdoknet/6358845.48701/33.918.374.757.143.317/figure-surface-bounded-labeled-changes-surface-element-surface.webp)
Inversion and Reflection
The Helmholtz Theorem
This result is valid for both static and time-dependent vector fields. 1.176) Substituting C(r) and∇2 in the last term and using the double curl identity in Example 1.3 gives. This follows from (1.76) and (1.78), which show that the divergence theorem transforms the volume integrals in the last two terms of (1.181) into the surface integrals.
Lagrange Multipliers
Sources, References, and Additional Reading
Problems
Levi-Civit`a Practice I
Levi-Civit`a Practice II Evaluate the following expressions which exploit the Einstein summation con- vention
Delta Function Identities A test function as part of the integrand is required to prove any delta function identity. With this in mind,
An Application of Stokes’ Theorem Without using vector identities, (a) use Stokes’ Theorem
Compute the Normal Vector Compute the unit normal vector ˆ n to the ellipsoidal surfaces defined by constant values of
A Variant of the Helmholtz Theorem I Mimic the proof of Helmholtz’ theorem in the text and prove that
Dot and Cross Products Let b be a vector and ˆn a unit vector
If you wake up a physicist in the middle of the night and say "Maxwell", he is sure to say "electromagnetic field".
Introduction
- Electric Charge
- Electric Current
- Conservation of Charge
For our purposes, the most useful statement of charge conservation begins with the surface-integral representation of the current Iin (2.7). The continuity equation states that the total charge in any infinitesimal volume is constant unless there is a net flow of pre-existing charge into or out of the volume through its surface.
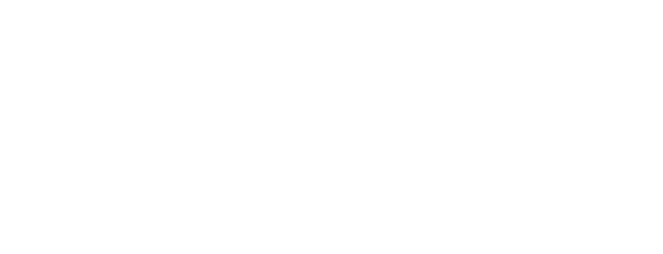
Moving Point Charges
- The Maxwell Equations in Vacuum
- Electrostatics
- The Field Concept
- Magnetostatics
- Faraday’s Law
- The Displacement Current
- Putting It All Together
- Microscopic vs. Macroscopic
- Lorentz Averaging
- The Macroscopic Surface
- Matching Conditions
- The Maxwell Equations in Matter
- Macroscopic Sources and Fields
- Microscopic Fields
- Quantum Limits and New Physics
- Quantized Matter
- Vacuum Polarization
- Quantum Fluctuations
- New Physics
- Magnetic Charge
- SI Units
- A Heuristic Derivation
- The Coulomb and Biot-Savart Laws The electric and magnetic fields for time-independent distributions of charge and current which go to zero at infinity are
- Time-Dependent Charges at Rest Consider a collection of point particles fixed in space with charge density ρ(r, t ) = !
- A Variation of Coulomb’s Law Suppose that the electric potential of a point charge at the origin were ϕ(r) = q
- Introduction
- The Scope of Electrostatics
- Coulomb’s Law
- The Scalar Potential
- The Coulomb Force is Conservative
- Matching Conditions for ϕ(r)
- Earnshaw’s Theorem
- Equipotential Surfaces and Electric Field Lines
- A Charged Line Segment
- Gauss’ Law and Solid Angle
- The Importance of Symmetry
- Matching Conditions for E(r)
- The Force on a Charged Surface
- Solid Angle
Maxwell characterized his theory as an attempt to "mathematize" the results of many different experimental investigations of electrical and magnetic phenomena. Another characteristic feature—the "spillout" of the electron distribution in the vacuum—becomes most apparent when we average ρ0(r) over planes parallel to . If the unit normal ˆn1 points outward from region 1 and the unit normal ˆn2 points outward from region 2 (Figure 2.8), the full set of matching conditions.
Maxwell's equations are a mathematical expression of known facts of experimental electromagnetism. Finally, SI eliminates ("rationalizes") the 4π factors in Maxwell's equations by introducing the magnetic constant μ0where. Example 3.2 The charge distributions in different atomic nuclei look very similar except for the change in extent.3 In Figure 3.2, ρA(r) is the charge density of the A nucleus and ifρB(r) is the charge density of the B nucleus.
The macroscopic adaptation conditions for the electrostatic potential follow from the behavior of the electric field near an interface with surface charge densityσ(rS) (Section 2.3.3). 68 ELECTROSTATICS: THE ELECTRIC FIELD PRODUCED BY STATIONARY CHARGE . the hole) with a surface charge density equal to the negative surface charge density of the shell.
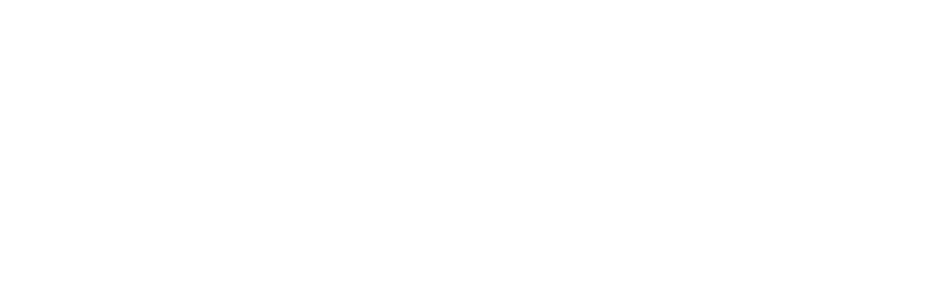
Field Lines for a Point Charge in a Uniform Field
- Electrostatic Potential Energy
- Coulomb Force from Variation of Potential Energy
- Green’s Reciprocity Relation
- Electrostatic Total Energy
- U E is Positive-Definite
- Interaction Total Energy is Potential Energy
It states that the potential energy of ρ2(r) in the field produced by ρ1(r) is equal to the potential energy of ρ1(r) in the field produced by ρ2(r). Instead of evaluating (3.76), we can calculate the work required to "build" the ball by successively adding spherical layers of uniform charge and thickness. A variation of the method used to obtain (3.77) can be used to derive (3.76) without going through the point load result (3.75).
3.78) Finally, it is worth noting that the total electrostatic energy UE is a quantity that clearly illustrates the fundamental limitations of the point charge concept in classical electrodynamics.UE. If qk= |rk| =1, numerical studies show that the energy of the minimum energy configuration is very well approximated by. Roughly, the first term is the average energy of N charges randomly distributed over the surface of the sphere.
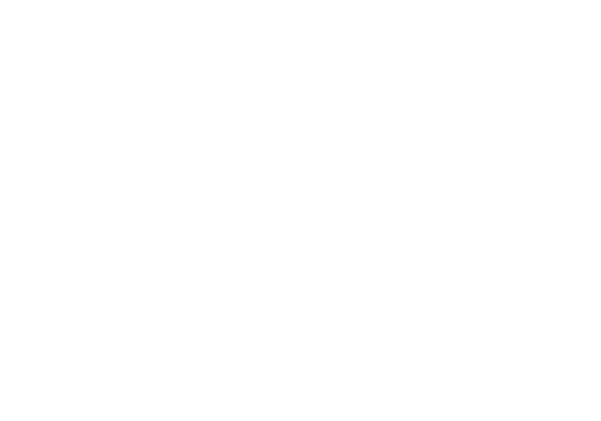
The Ionization Potential of a Metal Cluster
- The Electric Stress Tensor
- Applications of the Electric Stress Tensor
- Charged Particle Refraction
- Symmetric and Traceless The Cartesian components of the electric field in a charge-free region of space are E k = C k + D j k r j , where C k and D j k are constants
- Practice Superposing Fields This problem exploits the ring and disk electric fields calculated in Example 2.1
- Five Charges in a Line Draw the electric field line pattern for a line of five equally spaced charges with equal magnitude but alternating algebraic signs, as sketched below
- Gauss’ Law Practice Use Gauss’ law to find the electric field when the charge density is
- The Electric Field of a Charged Slab and a Charged Sheet (b) Find E(r) if ρ(x, y, z) = σ 0 δ(x) + ρ 0 θ(x) − ρ 0 θ(x − b)
- Two Electrostatic Theorems (a) Use Green’s second identity,
- Interaction Energy of Spheres
- Electrostatic Interaction Energy
- Ionization Energy of a Model Hydrogen Atom A model hydrogen atom is composed of a point nucleus with charge +| e | and an electron charge distribution
- Two Electric Field Formulae
- Introduction
- The Electric Multipole Expansion
- The Electric Dipole
- The Point Electric Dipole
- The Singularity at the Origin
- The Dipole Force
- The Dipole Torque
- The Dipole Potential Energy
- The Dipole-Dipole Interaction
- Electric Dipole Layers
- The Potential of a Dipole Layer
- Matching Conditions at a Dipole Layer
The electron is modeled as a negative point charge in the center of the sphere (black dot). With regard to the position of the electron, Gauss's law gives the electric field of the sphere as . To find the force on the charge atz= −d, we choose as the surface area of the massive cup-shaped object in Figure 3-18.
If both charges are counted, the component of the electric field is in the plane of the center line at point P. Using a side (edge) view, sketch the electric field lines everywhere assuming that the total charge of the disc is positive is. The matching condition,0nˆ2·[E1−E2]=σ(rS), tells us that the second term in (4.41) generates a jump in the normal component of the electric field atz=0.
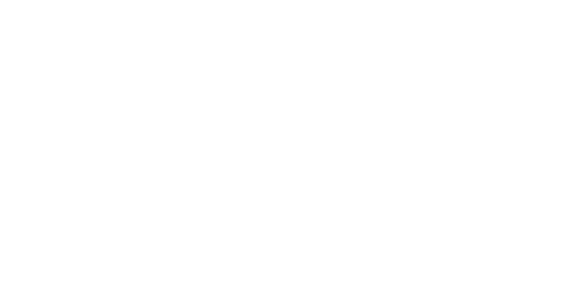
Monolayer Electric Dipole Drops
- The Electric Quadrupole
- The Traceless Quadrupole Tensor
- Force and Torque on a Quadrupole
The discontinuity (4.45) implies that the tangential component of the electric field is not continuous if the dipole layer density varies along the surface. The width w0 is independent of the droplet lengthL, as claimed in the problem statement. Repeating (4.6), the components of the electric quadrupole tensor are Qij Mimicking (4.9), it is not difficult to show that these scalars are uniquely defined and do not depend on the choice of the origin of coordinates if Q=p=0 .
9 The multiple derivative structure in (4.3) guarantees that each term in the multipole expansion can be represented by point charge configurations constructed from suitable pairings in space of the configurations describing the previous term. Therefore, a complete characterization of the quadrupole potential in an arbitrary coordinate system requires the specification of only five numbers (the independent components of the traceless Cartesian quadrupole tensor) instead of six numbers (the components of the primitive Cartesian quadrupole tensorQ). Following the logic of Section 4.2.3, it is not difficult to show that and (4.69) are valid for any neutral charge distribution with p=0 if the electric field does not vary too rapidly over the volume of the distribution.
Nuclear Quadrupole Moments
- Spherical Mathematics
- Legendre Polynomials
- Spherical Harmonics
- The Inverse Distance
- Spherical and Azimuthal Multipoles
- The Exterior Spherical Expansion
- The Interior Spherical Expansion
- Azimuthal Multipoles
- Preview: the Connection to Potential Theory
It would be much more convenient if the right-hand side of (4.78) were expressed explicitly in terms of spherical coordinates r=(r, θ, φ) andr=(r, θ, φ) defined with respect to a certain set of coordinates. axes in space. We derive multipole expansions of the electrostatic potential ϕ(r) suitable for systems with natural spherical symmetry by substituting (4.84) into When the charge density is localized and confined to the sphere of radiusRin Figure 4.1, the spherical analogue of the Cartesian expansion (4.3) which converges for observation points outside the sphere is. 4.86).
The Cartesian analogue of the inner expansion (4.89) is a power expansion of ϕ(x, y, z) around the origin of the coordinates. We need two properties for the spherical harmonics: 4.94) Substituting A mandB minto (4.86) and (4.89), respectively, and using (4.91) gives exterior and interior azimuthal multipole expansion in the form. This reflects the continuity of the electrostatic potential as the observation point passes through a charge layer.
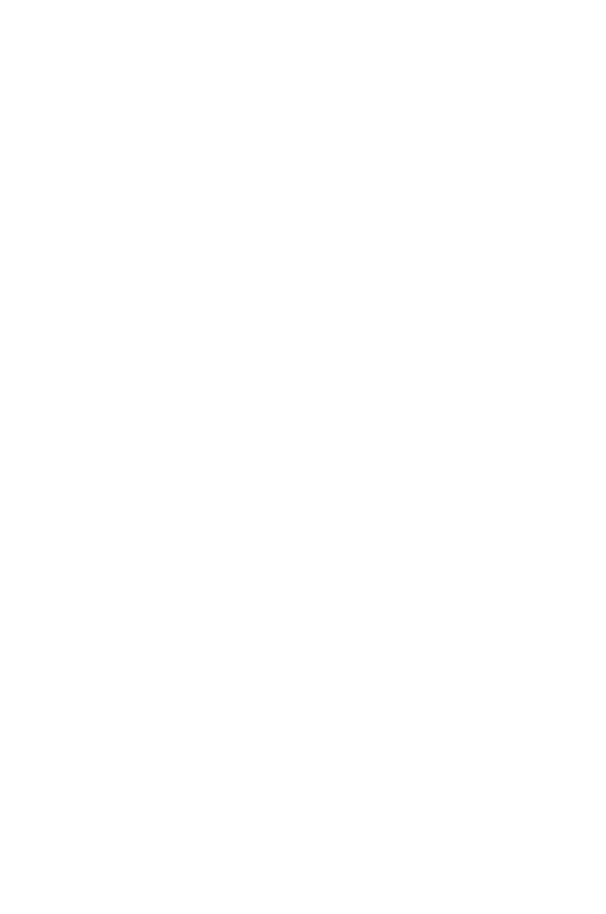
The Liquid Drop Model of Nuclear Fission
- Primitive and Traceless Multipole Moments
- Counting Multipole Moments
Assertion B: If U0 is the self-energy of the undistorted nucleus, the change in electrostatic energy caused by distortion of the form (4.101) is. There is a deep connection between the spherical multipole expansion derived in Section 4.6 and a traceless version of the Cartesian multiple expansion derived in Section 4.1.1, namely that the number of independent Cartesian multipole moments without trace Tij ..m() is equal to the number of spherical multipole momentsAmStarting at=2, the number in question is smaller (often much smaller) than the number of primitive independent Cartesian multipole moments,Cij ..m(). However, the term=2 is not the quadrupole potential (4.52) expressed in terms of primitive momentsQij.
4.125) The representation of the electrostatic potential by this traceless Cartesian expansion (4.123) is completely equivalent to the primitive Cartesian expansion (4.119). This means that the number of independent components of the tensor C()—call this number M—is equal to the number of ways in which each index takes one of the three values (x, y, z), regardless of their order. In the language of group theory, both representations of the potential are irreducible, that is, maximally efficient in our language.
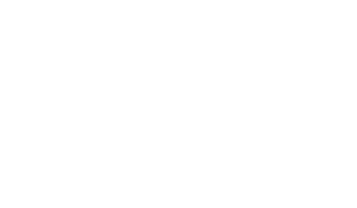
The Dielectric Polarization P(r)
- Dipole Moment Practice Find the electric dipole moment of
- Smolochowski’s Model of a Metal Surface A surprisingly realistic microscopic model for the charge density of a semi-infinite metal (with z = 0 as its macroscopic surface) consists of a positive charge
- The Charge Density of a Point Electric Dipole The text used Poisson’s equation to show that the charge density of a point electric dipole with moment p located at the point r 0 is ρ D (r) = − p · ∇ δ(r − r 0 )
- Stress Tensor Proof of No Self-Force Use the electric stress tensor formalism to prove that no isolated charge distribution ρ(r) can exert a net force on itself. Distinguish the cases when ρ(r) has a net charge and
- Potential of a Double Layer
- Interaction Energy of Adsorbed Molecules Molecules adsorbed on the surface of a solid crystal sur- face at low temperature typically arrange themselves into a periodic arrangement, e.g., one molecule lies
- The Many Faces of a Quadrupole
- Properties of a Point Electric Quadrupole
- Interaction Energy of Nitrogen Molecules How does the leading contribution to the electrostatic inter- action energy between two nitrogen molecules depend on the distance R between them?
- Foldy’s Formula The low-energy Born approximation to the amplitude for electron scattering from a neutron is proportional to the volume integral of the potential energy of interaction between the electron and
- Practice with Spherical Multipoles
- Exterior Multipoles for Specified Potential on a Sphere
- Analyze This Potential An asymptotic (long-distance) electrostatic potential has the form ϕ(r, θ, φ) = A
- Introduction
- Electrostatic Induction
- Thomson’s Theorem of Electrostatics
- The Surface Charge Density
- Screening and Shielding
- Capacitance
- Self-Capacitance
- What Does it Mean to ‘‘Ground’’ a Conductor?
- The Capacitance Matrix
- The Two-Conductor Capacitor
- The Energy of a System of Conductors
Pollock, "The calculation of the electrostatic energy in the liquid drop model of nuclear fission: A pedagogical note", Physica A. Find the angle α that minimizes the total energy and show that the energy/dipole is U= 1. b) The N2 molecule has a small quadrupole moment because covalent bonding builds up a negative (electron) charge in the bonding region between the atomic nuclei. Measurements of the electrostatic potential outside the box show that all the external multipole moments for = 1,2,. the electrostatic potential outside the box shows that all the external multipole moments for = 1,2,. is zero in a coordinate system with its origin at the center of the box.
Use the results of part (a) to find the asymptotic (r→ ∞) form of the potential produced by the shell. If there is source charge outside the conductor, the outer surface of the conductor develops a surface charge density. The conductor develops a surface charge density on the surface of the cavity and on the outer surface of the conductor.
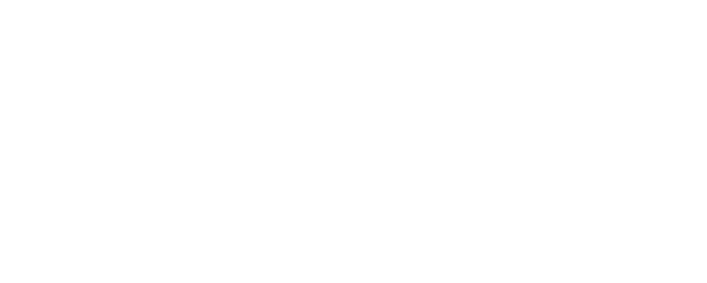
Coulomb Blockade
- Forces on Conductors
- Charges Held Constant
- Potentials Held Constant
- Why U E Differs From U ˆ E
- Real Conductors
- Debye-H¨uckel and Thomas-Fermi Screening
- A Dipole in a Cavity A point electric dipole with moment p is placed at the center of a hollow spherical cavity scooped out of an infinite conducting medium
- A Conducting Disk
- The Capacitance of Spheres
- Maxwell Was Not Always Right A non-conducting square has a fixed surface charge distribution. Make a rectangle with the same area and total charge by cutting off a slice from one side of the square and gluing
- Force Equivalence Confirm the assertion made in the text that the inverse relation between the matrix of capacitive coefficients and the matrix of potential coefficients implies the equivalence of these two
- Introduction
- Polarization
- The Volume Integral of P(r)
- The Lorentz Model
- The Modern Theory of Polarization
- The Field Produced by Polarized Matter
Also, there is no contribution to the force formula from the energy stored in the capacitor to the right of pointx+. The electric field is zero in the main part of the conductor (grey) except within a shielding layer (black) of thickness on the surfaces. Marcus, "The theory of the triode as a three-body problem in electrostatics", The American Physics Teacher.
Find the capacitance elements with potential difference ϕ2−ϕ1>0 as shown in the diagram below. To get a flavor of this approach, we recall from (6.4) that the dielectric charge density ρ(r) is the (negative) polarization divergence. However, if the polarization were simply the dipole moment per unit volume of charge density, P(r) would contain less information than ρ(r).
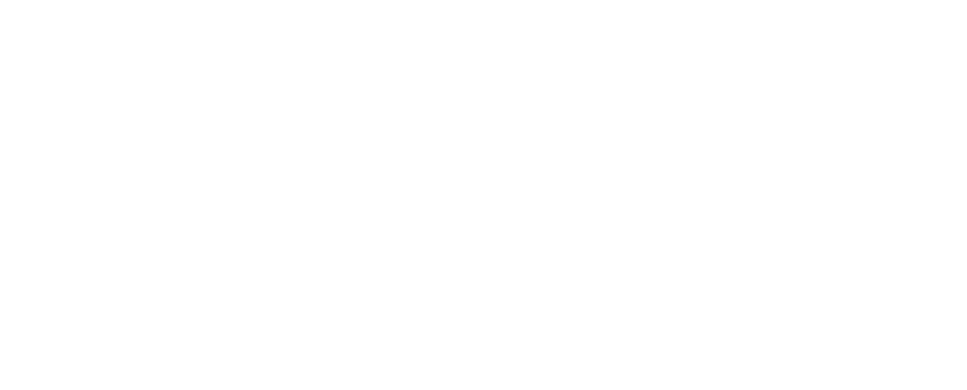
A Uniformly Polarized Sphere
- Polarized Matter as a Superposition of Point Dipoles
- The Total Electric Field
- The Auxiliary Field D(r)
- Matching Conditions
- Constitutive Relations
- Simple Dielectric Matter
- Fields and Sources in Simple Dielectric Matter
- Simple Dielectric Response to Free Charge
- Polarization Charge at a Simple Interface
- Simple Dielectric Response to Fixed Fields
The point dipole representation of the polarization qualitatively rationalizes the dependence of the polarization charge densities ρP(r) and σP(r) on P(r). The total electric field E in the Maxwell equations is the sum of the electric field EP produced by the polarization chargeρP(r)= −∇ ·Pand the electric field produced by all charges not associated with dielectric bodies. Gauss's law concerns the total charge, which is the sum of the polarization charge and the free charge.
Following the method of Section 2.3.3 (or otherwise), the matching conditions implied by (6.27) and (6.28) at an interface between two regions of space endowed with a surface density of free chargeσf. The electric field is reduced (shielded) compared to its value E0=zσ/ˆ 0 in the absence of dielectric. In other words, E=E0 when the dielectric is present because the charge flows from the battery (or whatever keeps the plates at a fixed potential) to the surface of the plates to exactly cancel the polarization charge on the adjacent dielectric surfaces.

Refraction of Field Lines at a Dielectric Interface
- Potential Theory for a Simple Dielectric
- The Physics of the Dielectric Constant
- The Electric Polarizability
- The Clausius-Mossotti Formula
- Polar Liquids and Solids
- The Limit κ → ∞
- The Energy of Dielectric Matter
If so, there must also be regions within the dielectric where the electric fields are smaller than (or opposite in direction to) the field of the vacuum capacitor. This is consistent with the discussion immediately above of a parallel plate capacitor with a fixed plate potential. The Lorentz model for polarizable matter (Section 6.2.2) relates the macroscopic dielectric constant κ [see (6.36)] of the dielectric gas to the microscopic polarizability α [see (6.75)] of the constituent atoms or molecules.
We also need an alternative definition of the cell-averaged dipole moment pR first defined in (6.10), namely,. On the other hand, the macroscopic fieldE(R) in (6.78) is the average of the field in a given cell from all sources, incl. This formula confirms the remark made at the end of Section 6.5.4 that the local electric field typically exceeds the average macroscopic field within a dielectric. Elocal(R) is due to all teoter molecules, so it is characteristic of the volume between gas molecules.
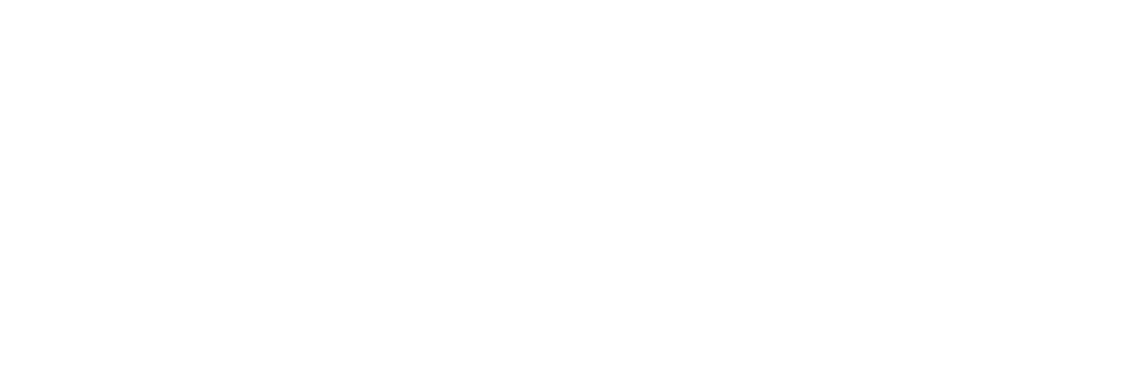