Preparation of Au/Ni electrode and morphology of Au/Ni electrode with different amount of Au. SEM images of the Au/Ni electrode (a) pristine, (b) after discharged for 110 cycles, and (c) after charged for 110 cycles. First 2 cycles of voltage profiles using Ag/Ni electrode on different electrolytes at 100 mAg-1Ag.
Introduction
Energy Storage System
The many combinations of anode, cathode, and electrolyte materials to produce different chemical reactions in cells produce numerous types of batteries from lead-acid, Li-ion to Li-S, and metal-air batteries.4 LIBs have been used the most common. type of battery for portable electronic devices due to its excellent cycleability and relatively high energy density.5 And since people need a more powerful battery with a much larger capacity, as the development of materials new for Li-ion batteries are mainly focused on increasing the specific capacity and reducing an overpotential during the charging process.6.
Lithium Ion Batteries
However, its poor conductivity is a problem so many coating methods have been applied to improve the low conductivity, but this leads to cost increase of the battery.9. The most commonly used as the cathode electrode material of the power battery can be spinel-based lithium ion; normally LiMn2O4 (LMO), Lithium Iron Phosphate (LFP), Nickel Cobalt Manganese (NCM), Nickel Cobalt Aluminum (NCA), and since the anode material is usually carbon and now the Lithium Titanium Oxide (LTO) to improve battery durability and performance of fast charging.6 Some of the current EV and the used batteries are listed in Table 1-1. Theoretically, lithium-air batteries can "at least" provide about 10 times the energy density - the amount of energy stored per kilogram - Current lithium-ion batteries can display about 200 kilowatts per kilogram.
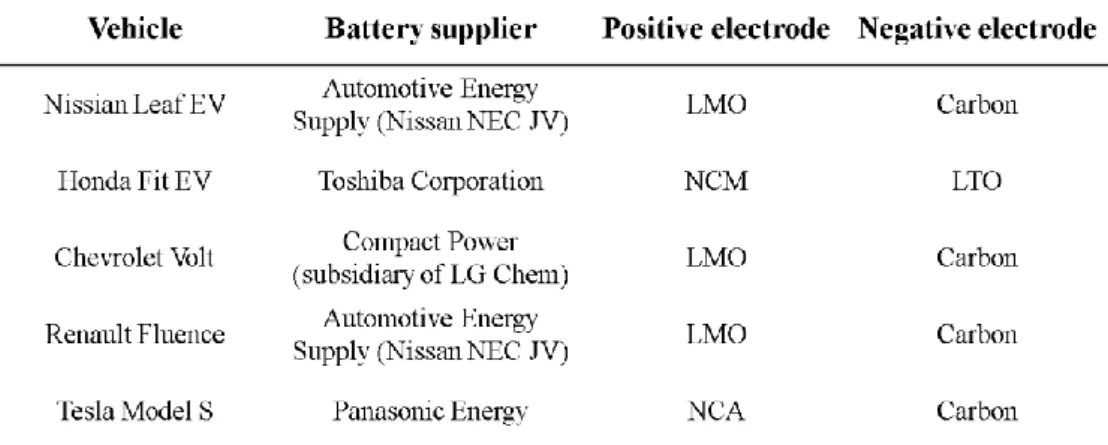
The Lithium Oxygen Batteries
ORR and OER
The maximum rate, observed for the platinum group metals in this case, requires intermediate values of ΔHf, with the rate being a combination of the rate of adsorption and the rate of desorption.29. Among them, the electrocatalytic performance (or reactivity) is the most essential and critical for the electrode reaction, and directly affects the overpotential of the OER. This means that there are many types of activation steps that control the rate of the OER.
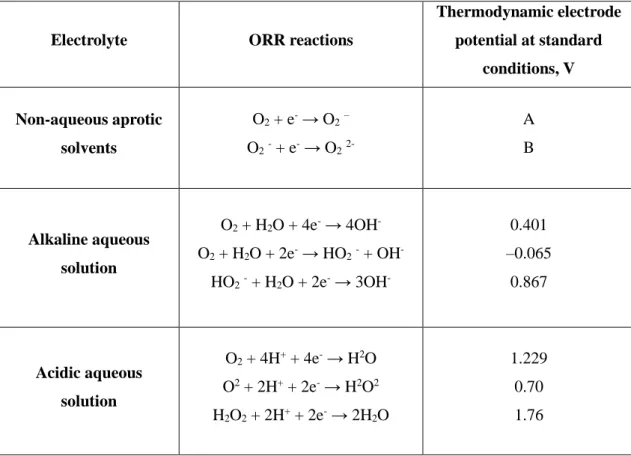
Review of catalysts for ORR and OER
The applied current and the capacity of the cells were calculated based on the amount of cathode material in the electrodes. SEM images of the as-fabricated (a) Ni nanowire, (b) Au/Ni electrode, TEM images of (c) Ni nanowire, (d) Au/Ni electrode, and EDX images of (e) Ni, and (f ) ) Au element respectively. XRD patterns of the cycled Au/Ni electrode were also not changed compared to the pristine one.
A clue to the origin of the performance fading can be further explained by examining the SEM images of Li metal (Figure 3-17). In addition, the Li-O2 cell composed of Au/Ni electrode can withstand excellent reversible cycling for more than 200 cycles (including the regenerated cell test). SEM images of fabricated (a) Ni nanowire, (b) Ag/Ni electrode, TEM images of (c) Ni nanowire substrate, (d) Ag/Ni electrode and EDX images of (e) Ni and (f) Ag element or
Discharge capacities and cycle performance of the Ag/Ni electrode were greatly affected by the electrolytes. From their study, it is clear that the decomposition of the DMSO solvent cannot be avoided at the Ag/Ni electrode. Moreover, the Ag peak intensity in Figure 4-4d was comparable to that of pristine Ag/Ni electrode, unlike the results of the other electrolytes.
R.; Urbonaite, S.; Bjorefors, F.; Edstrom, K., Influence of the cathode porosity on the discharge performance of the lithium-oxygen battery.
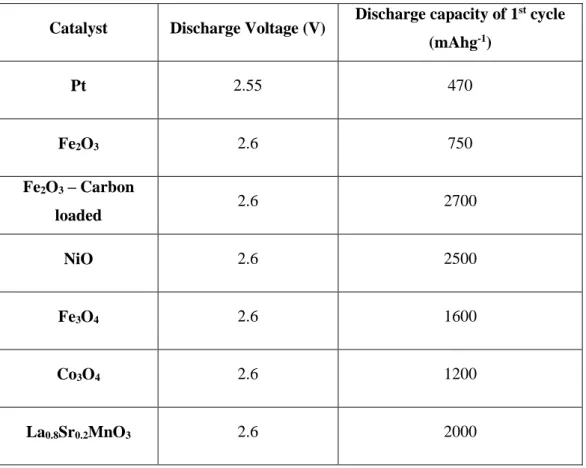
The Zn-air battery
The Li-O 2 battery
The Li-O2 battery (often called Li-air or lithium-air battery) is the battery that, like other Zn-air batteries, consumes oxygen from the ambient atmosphere. In addition, the Li-O2 battery possesses a very high specific energy of approximately 3700 mAh/g, because lithium is the lightest metal, which is much greater than that of graphite or other commercially available anodes (including other metal air batteries, of course). The common point is that the Li-O2 and Zn-air battery both react with reduced oxygen.
Therefore, the researches so far have mostly investigated the Li-O2 system using pure oxygen gas.75 In the Li-O2 battery with a non-aqueous electrolyte (the Li-O2 battery can be divided into four types on based on the electrolyte type, shown in Figure 2-10 but in this thesis I focused on non-aqueous electrolyte systems), reactions between reduced oxygen and lithium ions result in the formation of lithium peroxide (Li2O2) (or possibly lithium oxide (Li2O)) as the final discharge reaction product on or in the cathode pores or surfaces. Since the capacity of the Li-O2 battery can be determined by the total amount of Li that can be stored in Li2O2 or Li2O in the cathode, the capacity of the Li-O2 battery is normalized according to the cathode weight and is preferred given to porous materials as cathode. The non-aqueous Li-O2 battery was first reported by Abraham and Jiang in 1996.76 Since then, tremendous efforts have been made to improve the performance of the Li-O2.
The cathode material for the Li-O2 battery is usually a porous carbon, which can store more discharge products. At the early stage of the Li-O2 battery (around ~2010), several studies have been mainly focused on carbon and catalysts to increase the discharge capacity or to optimize the properties of the carbon cathode.33c, 80 Most of these studies aimed to to improve the cathode formulation to provide more space to store larger amounts of Li2O2. Many variable factors are interrelated, such as carbon pore size distribution (PSD), pore volume, porosity, the electrode thickness, the loading density, the cathode formulation, etc., that affect the discharge capacity and performance of the Li-O2 battery. 48a, 81.
I mentioned above the problems with the carbon cathode and the breakdown or degradation of the electrolytes and salts in the Li-O2 battery.
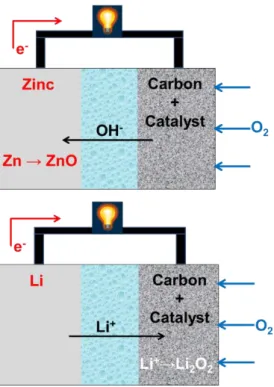
Experiment Ⅰ
The Cell Setup
- Introduction
- Experimental Section
- Results and Discussion
- Conclusion
For electrochemical characterizations, the Au sheet electrode and the Au/Ni electrode were cycled between 2.3 and 4.3 V at different current conditions. Before the electrochemical test of the full cell with the Au/Ni electrode, the stability of the Au/Ni electrode was confirmed by a cyclic voltammetry (CV) test. A whole-cell test of Au/Ni electrode with different amount of Au was performed at a current rate of 500 mAg-1Au in 1.3 M LiTFSI in TEGDME (tetraethylene glycol dimethyl ether) electrolyte under 1 atm O2 blowing at 24 oC.
The discharge capacity of the Au/Ni electrode is far superior to that of the Au leaf at various currents, and for example, the discharge capacity of Au/Ni was 921 mAhg-1Au, while the discharge capacity of the Au leaf was 134 mAhg-1Au. Furthermore, the cyclic performance of the Au/Ni electrode was performed over 100 cycles, and the capacity of the Au/Ni electrode was 591 mAhg-1Au and 580 mAhg-1Au after the 1st and 100th cycle at 500 mAhg-1Au. To investigate the stability of elements and surface morphological change of Au/Ni electrode, XPS (Figure 3-12), SEM (Figure 3-13) and XRD (Figure 3-14) were investigated.
SEM images show that the Li2O2 was covered with the pores of the Au/Ni electrode after full discharge, but the blocked pores were almost recovered after charging. The morphology of the Au/Ni electrode is almost unchanged compared to the pristine one shown in Figure 3-13. 1 dis. a) Raman and (b) FT-IR spectra of Au/Ni electrode, Li2O2 and Li2CO3 Both spectra, however, are too noisy to assign.
After the 110th cycle, a vividly developed black layer was observed, indicating that the capacity fading is not due to the Au/Ni electrode, but to the Li anode. We have designed a highly efficient Au/Ni electrode using a simple electrodeposition method for Li-O2 battery, which results in improved cycling stability and improved capacity compared to nanoporous Au sheet. We confirm that the capacity fading was due to Li metal passivation due to the electrolyte degradation (TEGDME), not the Au/Ni electrode.
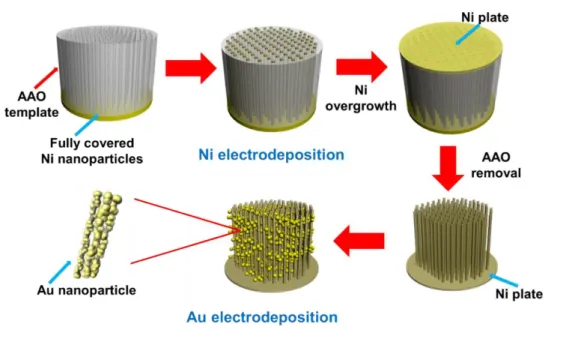
Experiment Ⅱ
A Possibility of Silver catalyst as a Carbon- and Binder-free Cathode material
- Introduction
- Experimental Section
- Results and Discussion
- Conclusion
The SEM images revealed that a severe passivation layer covered all the pores of Ag/Ni electrode only after 2 cycles between 2.3 V and 3.8 V. The morphology of Ag/Ni electrode in NMP/1M LiTFSI is also almost unchanged compared to the pristine one, as shown in Figure 4-3. The DME-based electrolyte severely evaporated from a cell during cycling due to its high volatility and electrochemically decomposed at the Ag/Ni electrode.
The Li 1s, F 1s (Figure 4- 4a and c) and S 2p (Figure 4-5) XPS spectra further confirmed that the LiTFSI salt and ether solvents undergo electrochemical decomposition at the Ag/Ni electrode. This thick surface layer blocks the Ag signal from the Ag/Ni electrode and thus the Ag peak intensity in Figure 4-4d was extremely lower than that of pristine Ag/Ni electrode. We could conclude from the SEM images in Figure 4-3 and XPS data in Figure 4-4 that the NMP/1M LiTFSI has relatively better stability compared to other electrolytes in Li-O2 cells with the Ag/Ni electrode .
However, as mentioned above, the decomposition of LiTFSI salt cannot be avoided in ether-based electrolytes in Li-O2 cells with Ag/Ni electrode. From these results, it is believed that NMP/1M LiTFSI electrolyte is suitable for Ag/Ni electrode in Li-O2 battery. Consequently, to further verify the performance of the Ag/Ni electrode with NMP, we performed more cycle tests shown in Figure 4-8.
This indicates that the Ag/Ni electrode with NMP/1M LiTFSI electrolyte has relatively good cycling stability in initial cycles, but NMP solvent decomposition eventually occurs during longer cycling.
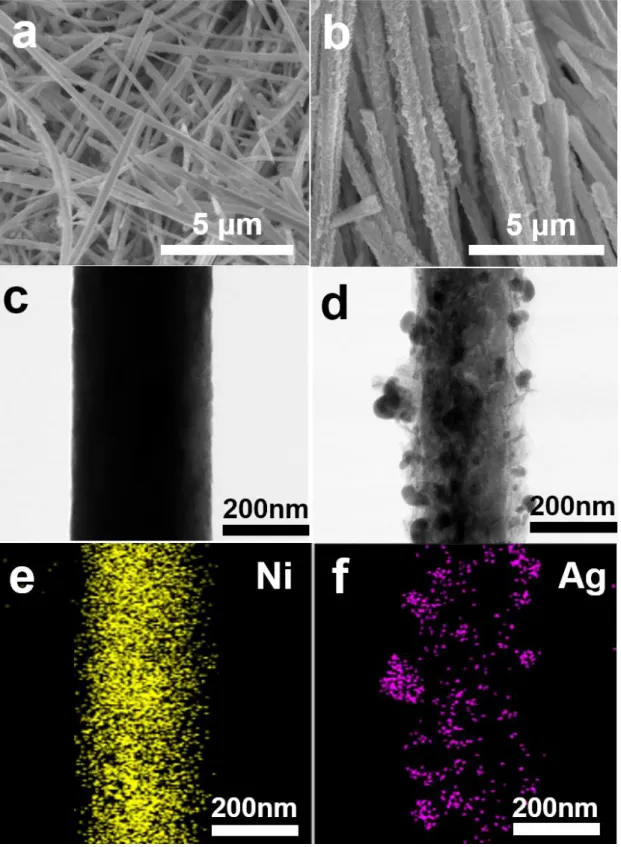
A.; Solorza-Feria, O., Electrocatalysis of oxygen reduction on carbon-based Ru-based catalysts in a polymer electrolyte fuel cell. Zelenay, P., Nitrogen-doped graphene-rich catalysts derived from heteroatom polymers for oxygen reduction in nonaqueous lithium-O-2 battery cathodes. A.; Amine, K., Increased stability toward oxygen reduction products for lithium-air batteries with oligoether-functionalized silane electrolytes.
E.; Bernhard, J.; Kaiser, U.; Wohlfahrt-Mehrens, M.; Jorissen, L., Au-coated carbon cathodes for enhanced oxygen reduction and evolution kinetics in aprotic Li-O-2 batteries. K.; Scrosati, B., An improved high-performance lithium-air battery. a) Mizuno, F.; Nakanishi, S.; Kotani, Y.; Yokoishi, S.; Iba, H., Rechargeable Li-Air batteries with carbonate-based liquid electrolytes. A.; Shao-Horn, Y., Method development to evaluate the oxygen reduction activity of high surface area catalysts for Li-Air batteries.
Acknowledgement