Isocontours temperature and different species for self-igniting laminar lifted methane/hydrogen jet flames under HTLH condition. Isocontours temperature and different species for self-igniting laminar lifted methane/hydrogen jet flames under LTHH condition.
Introduction
While in a previous experimental study of self-ignited laminar methane/hydrogen jet flames [24], an unusual variation in launch altitude with U0 was observed, or HL decreases with increasing U0 at relatively low inlet temperatures and relatively high hydrogen content; the decreasing HL with U0 does not follow the conventional self-ignited laminar liftoff height behavior of 𝐻L ~𝑈0 [24]. Therefore, the present study will also investigate the characteristics of self-ignited laminar raised flames with MILD combustion.
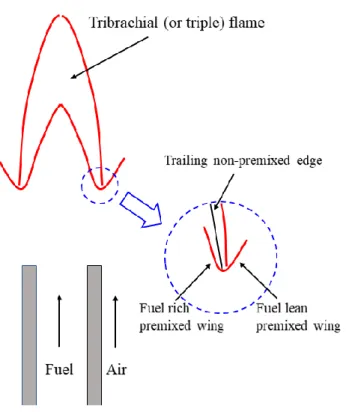
Numerical methods
Schematic of the computational setup for the present simulations of self-igniting laminar lifted methane/hydrogen jet flames in heated coflow. A detailed analysis of the flame stabilization of self-igniting laminar lifted methane/hydrogen jet flames will be made in the next section. Unlike the cases of normal 𝐷H2, HL increases with increasing U0, which clearly shows the effect of 𝐷H2 on the lifting properties of the lifted methane/hydrogen jet flames.
6, the radial location of the flame base moves from the center for the MILD combustion regime to the radially outer position for the tribrachial edge flame regime. These auto-ignition characteristics imply that the HL of the lifted methane/hydrogen jet flames under the LTHH condition would decrease with increasing U0, provided that their stabilization mechanism at the flame base is auto-ignition. In particular, the contribution of the chain branching reaction (R35) and the hydrogen heat release reaction of H2 + OH → H + H2O (R79) become dominant just upstream of the flame base for the tribrachial edge flame regime.
In the tribrachial edge flame regime, the total contribution of hydrogen-related reactions to the CEM becomes larger than that in the LIGHT combustion regime, leading to a decrease in the flame-based ignition delay for the tribrachial edge flame regime.
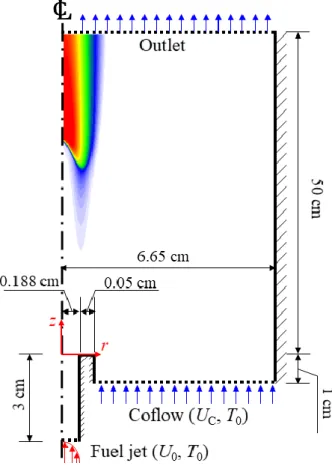
Overall characteristics of lifted flame
Autoignited laminar lifted flame under HTLH condition
The lifted flame attaches to the fuel nozzle when U0 = 10 m/s or less, which is qualitatively consistent with a previous experimental observation [24]. The shift of the flame base to a very lean mixture (𝜉fb < 0.1) means that the stabilization mechanism of self-igniting laminar lift flames would be different from that of non-self-igniting laminar lift flames where the flame base coincides with the point of the stoichiometric mixture line where the flame edge is. Although the raised flame stabilizes at lean fuel mixture, the peak temperature and OH mass fraction occur along the stoichiometric mixture line in the region downstream of the flame base, similar to previous numerical results.
4b and 4c show that these intermediate species reach their maximum values upstream of the flame region, implying that autoignition would be predominant in the stabilization of the lifted flame. The general characteristics of the self-ignited laminar lifted flame under HTLH conditions are summarized in Figure 5, which shows HL and (Tmax-T0)/Tig variations with the different U0, where Tmax is the maximum flame temperature in the domain, and Tig the minimum auto-ignition temperature of the stoichiometric mixture based on the inlet conditions. 5 aims to identify the combustion mode of the raised flames between the lifted flame with tribrachial edge and lifted flame with mild combustion.
It can be easily seen in Figure 5 that (Tmax−T0)/Tig is above unity for all U0s, indicating that only a raised flame with a tribrachial edge occurs under the HTLH condition.
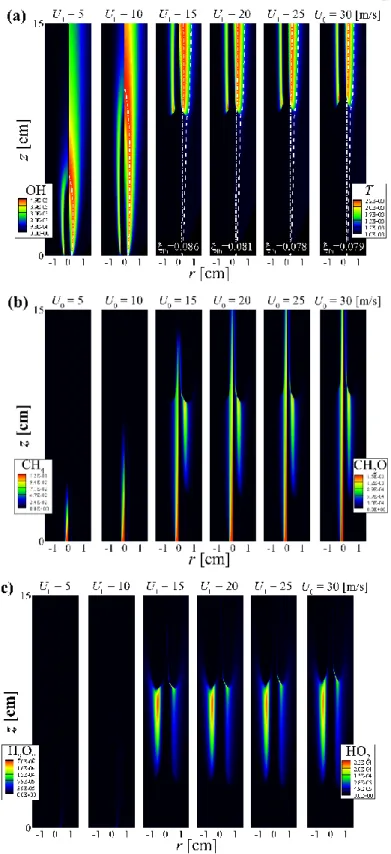
Autoignited laminar lifted flame under LTHH condition
In the auto-ignition of various types of hydrocarbon fuels, CH2O, HO2 and H2O2 are known to be important intermediate species during the early stage of auto-ignition process, as they are characterized by their concentrations peaking before auto-ignition occurs. Second, figures 6(b) and 6(c) show that intermediate species such as CH2O, HO2 and H2O2 are predominant at the upstream of flame base for all U0 cases, indicating that the self-ignition affects the stabilization of the lifted flames. From these results, we can suspect that the unusual decreasing behavior of HL under LTHH condition will be attributed to the characteristics of self-ignition and MAGTE combustion, which depends on the hydrogen content in the fuel jet.
As in the HTLH regime, we define the base of the flame under the LTHH condition as the highest point of the YOH isoline, which is approximately 5% of the maximum value in the domain. To clarify the exact location of the base of the flame in MILD combustion, we therefore adopt Chemical Explosive Mode Analysis (CEMA) and define the base of the flame as the uppermost location of the Re(𝜆exp) = 0 isoline, where 𝜆exp is the eigenvalue of the Jacobian of the chemical source term. To confirm such a definition of the flame base, the flame base defined by YOH and 𝜆exp = 0 is compared with the tribrachial edge flame, resulting in almost the same location of the flame base between the two definitions, as shown in Fig. 8 .
It is noted that both isolines are close to where the heat release rate is maximum in the domain and maximum YOH is lower than for the MILD combustion case (ie U0 = 4 m/s).
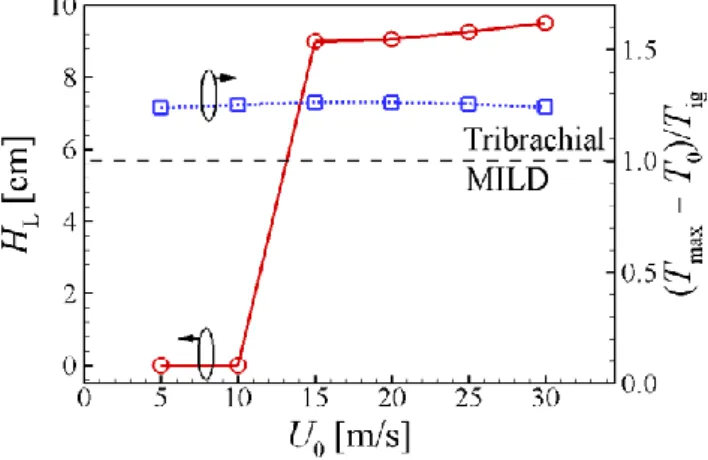
Hydrogen effect on H L and ignition
We first convert the mixture at the flame base to unburned components of CH4, H2, O2 and N2 using the law of conservation of elements. Ignition delay, τig,fb, and hydrogen ratio, RH,fb, based on the mixture state at the flame base as a function of the fuel jet velocity U0 under the LTHH condition (T0 = 950 K and RH = 0.30). However, for the MILD combustion regime, hydrogen molecules diffuse out from the center and therefore only methane molecules remain at the flame base, especially for very low U0.
The ignition delay in the center becomes shortest and the MILD combustion has a flat or “U” shaped structure depending on the axial velocity profile. Moreover, small 𝐷H2 makes the mixture at the center relatively more fuel-rich than that with large 𝐷H2, and therefore for the MILD combustion regime, the HL with modified 𝐷H2 becomes shorter than that with normal 𝐷H2, and vice versa for the tribrachial edge flame regime, as shown in Fig. 10. Thus, it is reasonable that the launch altitudes with normal and modified 𝐷H2 almost cross at the boundary between the tribrachial edge flame and the MILD combustion regimes or at the transition regime characterized by (Tmax−T0)/Tig ≈ 1.
If Da < 1 at all the points upstream of flame base, it indicates that the time for the mixtures to self-ignite (𝜏ig) takes longer than the time to reach the flame base from the point (𝜏flow).
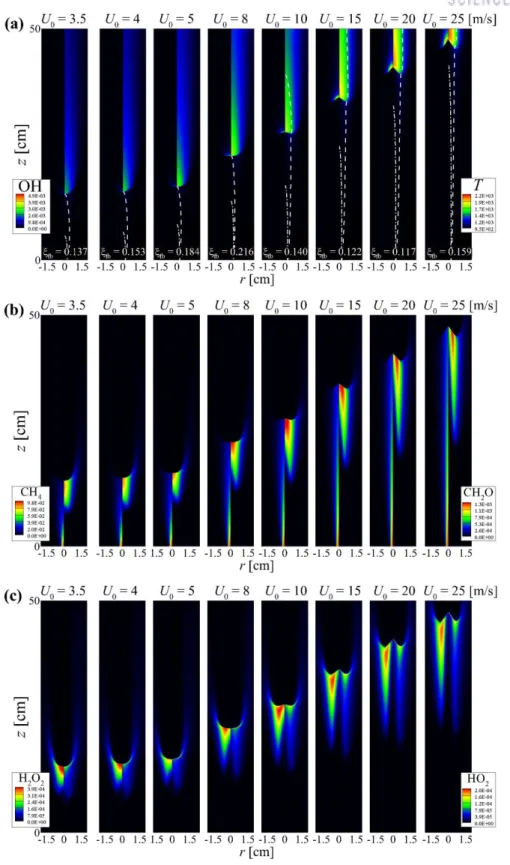
Ignition characteristics: CEMA
First, for all regimes the most important EI variable upstream of the flame base is temperature, while CH3, CH2O, and HO2 also contribute to CEM higher than the flame base, indicating a general methane/air autoignition sequence [55]. Third, for the tribrachial flame edge regime, the contribution of hydrogen to the CEM is identified as the second largest after the temperature just ahead of the flame base, while its contribution is negligible for the MILD combustion regime. To further identify the chemical characteristics of elevated flames in different regimes, the contribution of each chemical reaction to the CEM or PI isocontour of significant reactions is shown in Fig. 15 .
Although we have already had a glimpse of the chemical characteristics of the lifted flames through the EI analysis, we can further investigate which reaction affects the CEM through the PI analysis. The final CO conversion to CO2 appears as the most important heat release step right after the conversion of HCO to CO which occurs just upstream of the flame as described in Fig.15(c) and (d). It is important to note that from the EI isocontours of the lifted flames with modified 𝐷H2 as shown in figure 16, their chemical characteristics are found to be very similar to those of the lifted flames with normal 𝐷H2 in the transition regime (i.e. the case with U0 = 8 m/s).
As a result, their chemical characteristics become similar to those of raised flames with normal 𝐷H2 in the transition regime, in which the effect of large 𝐷H2 on the ignition of the methane/hydrogen jet becomes minimal.
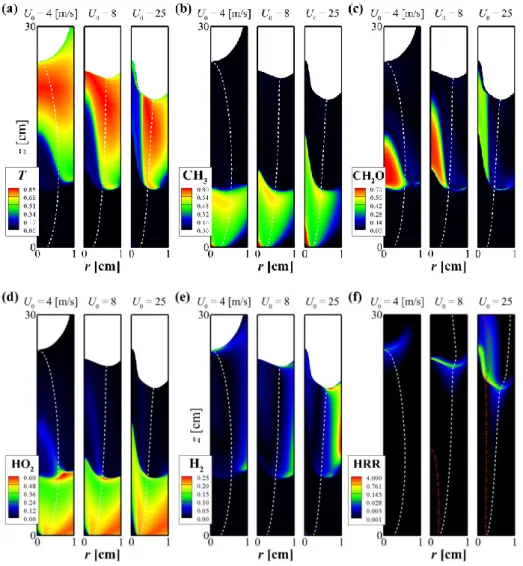
Liftoff height correlation
It is easily seen from the figure that the new 𝐻L correlation accurately captures the decreasing behavior of 𝐻L with increasing 𝑈0, confirming that the refined 𝜏ig,fb reasonably well represents the ignition delay of the self-igniting laminar lifted methane/hydrogen, although it does not can beam be obtained in advance. It is also interesting to note that the conventional lift-height correlation of 𝐻L ~ 𝑈0𝜏ig,st2 applies to self-igniting single-fuel laminar lifted jet flames because for these flames 𝜏ig,fb remains almost the same for different U0 as shown in Fig. 11 and replacing 𝜏ig,st with 𝜏ig,fb may not affect the liftoff height correlation.
This can be attributed to the uncertainty of the exact location of the flame, and as future work, the autoignition characteristics of the very MILD combustion regime should be examined.
Conclusions
Law, Direct numerical simulations of ignition of a lean n-heptane/air mixture with temperature inhomogeneities at constant volume: Parametric study, Combust. Chen, A DNS study of ignition characteristics of a lean iso-octane/air mixture under HCCI and SACI conditions, Proc. Yoo, Direct numerical simulations of ignition of lean primary reference fuel/air mixtures with temperature inhomogeneities, Combust.
Yoo, Direct numerical simulations of lean biodiesel/air mixture ignition with temperature and composition inhomogeneities at high pressure and intermediate temperature, Combust. Yoo, A DNS study on the ignition of lean PRF/air mixtures with temperature inhomogeneities at high pressure and intermediate temperature, Combust. Yoo, Direct numerical simulations of lean n-heptane/air mixture ignition with temperature and composition inhomogeneities relevant to HCCI and SCCI combustion, Combust.
Yoo, Ignition of a lean PRF/air mixture under RCCI/SCCI conditions: A comparative DNS study, Proc.