This book is not intended to be a reference book that summarizes and evaluates all of the reactions and phase transformations relevant to MSE, although some unique topics are presented. Fifty years ago, all students in this particular program would have been interested only in metals, but not today. This typically serves as a first introduction to nuclear chemistry for over 80% of students, despite its importance.
HOW THIS BOOK DEVELOPED
This book explicitly shows how to achieve this result without more than preliminary calculation and serves as an example of one of the several kinetic concepts an undergraduate MSE student should be exposed to before entering the materials profession as a researcher or practicing engineer. In one of the author's teaching experiences where about 100 MSE students took the kinetics course, over 50% were interested in soft materials. With the growing importance and interest in soft materials - with a significant interest overlap with chemistry, physics, chemistry and biotechnology -.
THE TARGET AUDIENCE
However, for soft materials such as polymers, it is more challenging to easily match the kinetics of processing of hard materials. First, the useful temperature range for polymers is only a few hundred degrees Celsius, while hard material processing covers a range of more than 3000°C. Second, the microstructure of hard materials can be greatly varied, largely controlled by kinetic processes at elevated temperatures, the main factor determining properties.
HOW THE BOOK IS ORGANIZED
That is why MSE exists: commonality in the processing, structure and properties of materials. In contrast, it is the chemistry, length and entanglement or cross-linking of polymer molecules that have the greatest influence on polymer properties.
SUMMARIZING THE MAIN FEATURES OF THIS BOOK
However, a course in differential equations is not a requirement because most equations can be solved easily, usually by separation of variables, so a second calculus course will suffice. Chapter 1 provides a brief overview of the essentials of thermodynamics that are important in later chapters of this book. Likewise, parts of chapters 13 through 16 may be included depending on the background of the students and the intent of the course.
MATHEMATICS
UNITS, GRAPHS, AND PLOTS
Kinetics and Materials Science and Engineering 3
- Historical Development of MSE as a Discipline 6
- Why Thermodynamics Is Difficult 10 1.6.2.3 Significant Events in the Development
- Standard States and Equilibrium Constants 18 1.7.6.3 Gibbs Energy as a Function of Temperature 20
- INTRODUCTION
- MATERIALS SCIENCE AND ENGINEERING
- Chemical Potential and Partial Molar Gibbs Energy 24
- Phase Diagrams and Gibbs Energy-Composition Diagrams 29
- HISTORICAL DEVELOPMENT OF MSE AS A DISCIPLINE
- IMPACT OF MSE
- MICROSTRUCTURE
- THERMODYNAMICS REVIEW .1 A D ifficult S ubject
- H iStory of t HermoDynAmicS .1 Relation to MSE
- REVIEW OF THERMODYNAMIC CONCEPTS .1 P urPoSe
- f our l AwS of t HermoDynAmicS
- f irSt l Aw of t HermoDynAmicS
- S econD l Aw of t HermoDynAmicS
- H elmHoltz AnD G ibbS e nerGieS
- r eActionS AnD e quilibrium c onStAntS
- m ulticomPonent S yStemS
- P HASe r ule AnD P HASe D iAGrAmS
- CHAPTER SUMMARY
The second law of thermodynamics states that the entropy of the universe increases during a process. V is the molar volume of the mixture or solution and the mole fraction Xi is simply Xi=ni (nA+nB). Again, to the left of the two-phase α + liquid region (point 1) the single phase α has the lowest energy and is the stable phase.
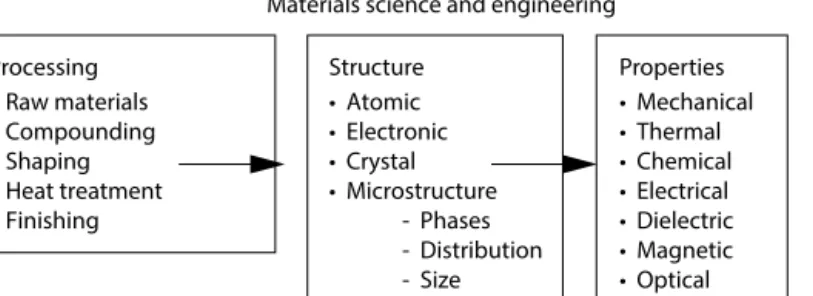
APPENDIX
For compositions to the left of the point of tangency 1 on the α-phase, the α-phase has the lowest Gibbs energy and so this point of contact determines the maximum composition of single-phase alpha on the phase diagram in Figure 1.16. Again, the extensions of the tangents to the vertical axes give the activities (actually RT ln a) of A and B for the compositions of the three phases at the tangents. Between points 1 and 2, the tangent line has the lowest energy, so these two points give the limits of the two-phase α + β region.
- How Good Is Stirling’s Approximation?
- Molar Entropy of Mixing of Two Crystalline Elements
- Molar Entropy of Mixing of Two Compounds
The mixing entropy of an ideal solution can be modeled by the Boltzmann equation for the entropy S k= BlnW. Therefore, the ideal molar entropy of the mixture of these two compounds is also given by equation A.6 since the two cations are distributed over a. However, what is not always appreciated is that the molar entropy of the mixture is different if the two components are Al2O3 and Cr2O3 which also form a near-ideal solid solution.
EXERCISES
In this case, the total number of sites over which the aluminum and chromium ions are distributed is not NA but 2NA since there are two cations for each mole of the two constituent compounds. Calculate the equilibrium partial pressures of dichlorosilane and HCl over silicon at 1400 K if the initial pressures are p(SiH2Cl2) = 0.01 atm and p(HCl) = 0. If the Gibbs energy of formation of the compound AB is −120 kJ/mol at the AB/0 in the thermodynamic composition at T4, calculate the activity of 4o in the total B at T4.
Introduction to Kinetic Processes in Materials 41 Chapter 3 Second-Order and Multistep Reactions 61 Chapter 4 Temperature Dependence of the Reaction Rate Constant 95
- Introduction: Material Transport and Reaction Rates 42 .1 Example: Deposition of Silicon from the Gas 42
- Example of First-Order Reaction: COCl 2 Decomposition 52 2.6 Radioactive Decay and Related Nuclear Reactions 52
- INTRODUCTION: MATERIAL TRANSPORT AND REACTION RATES
- E xamplE : D Eposition of s ilicon from thE G as
- Importance of First-Order Reactions in Materials 57
- E xamplE : D issolution r atEs of n a c l anD a l 2 o 3 , c ontrast D iffusion anD r Eaction c ontrol
- c onclusions from E xamplEs
- HOMOGENEOUS AND HETEROGENEOUS REACTIONS
- HOMOGENEOUS REACTION RATES .1 W hy s tuDy ?
- r Eaction o rDEr
- u nits anD s ymbols
- ZERO-ORDER REACTION
- FIRST-ORDER REACTION .1 i ntEGratED E quation
- f raction r EactED
- t hE h alf -l ifE of a r Eaction
- r Elaxation t imE
- E xamplE of f irst -o rDEr r Eaction : coc l 2 D Ecomposition
- RADIOACTIVE DECAY AND RELATED NUCLEAR REACTIONS .1 i DEal f irst -o rDEr r Eaction
- u ranium i sotopEs
- r aDioisotopE p oWEr s ourcEs
- n uclEar r Eactors anD f ission p roDucts
- r aDiocarbon D atinG
- IMPORTANCE OF FIRST-ORDER REACTIONS IN MATERIALS
- CHAPTER SUMMARY
- First-Order Reaction That Goes to Equilibrium and Not Completion 66 3.4 Second Order ⇔ Second Order That Goes to Equilibrium 68
- INTRODUCTION
- SECOND-ORDER REACTIONS .1 O ne R eactant
- t wO R eactants
- P seudO F iRst -O RdeR R eactiOn
- FIRST-ORDER REACTION THAT GOES TO EQUILIBRIUM AND NOT COMPLETION
- SECOND ORDER ⇔ SECOND ORDER THAT GOES TO EQUILIBRIUM
- G eneRal
- e quilibRium
- s PeciFic e xamPle
- t he G eneRal s OlutiOn
- n umeRical s OlutiOn
- PARALLEL AND SERIES REACTIONS .1 i ntROductiOn
- P aRallel R eactiOns
- s eRies R eactiOns
- COMPLEXITY OF REAL REACTIONS .1 h ydROGen i Odide
- c hain R eactiOns
- c hain P OlymeRizatiOn .1 Mechanism
- c hain b RanchinG R eactiOns
- SUMMARY
- Initial Concentrations Equal
- Finding Roots (Factors)
- Checking the Factoring
- Checking the Solution
This reaction step is followed by diffusion of solute ions away from the surface. The dissolution rate of NaCl is generally controlled by liquid diffusion of solute ions away from the surface. This reaction rate is extremely small, despite the fact that the thermodynamics for dissolution are quite favorable.
In all cases, the driving force for the reaction is a reduction or minimization of the Gibbs energy of the system. So the order of the reaction leads to different expressions for the concentrations as a function of the experimental variables, time and concentrations. Note also the very large value of Q (345 kJ) that produces the very strong temperature dependence of the reaction rate constant.
In this case, the reaction rate is proportional to one of the reactants with the reaction rate constant k, the constant of proportionality. This is a very general result: for parallel reactions, the fastest (fastest if more than two products) controls the reaction rate. Consider a series reaction A →k1 B →k2 C, where the slower of the two reactions—the smaller of k1 and k2—determines the overall rate, or [C](t).
In Appendix A.3.5 it is shown that it is the smaller of the two "ks" - the slower reaction - that controls the overall reaction rate. Where can data on gas phase reaction kinetics be found?” The answer to the second question is that the National Institute of Standards and Technology (NIST) of the USA. Up to this point, little has been said about the temperature dependence of the reaction rate constant.
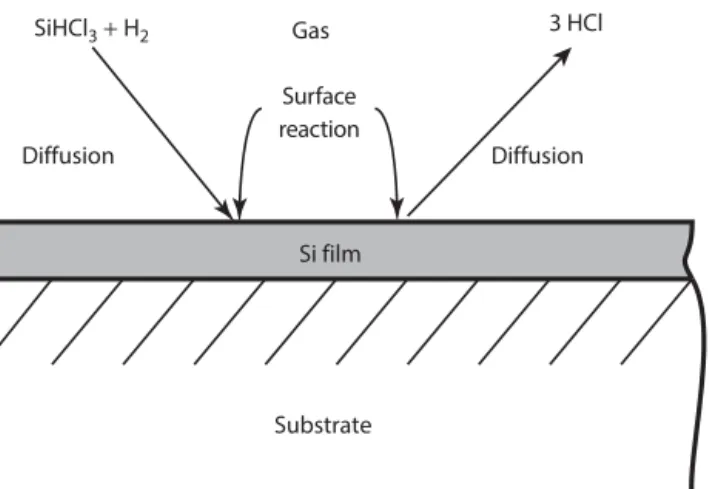
Introduction
When t → ∞, only the first term in the numerator remains and it is x1 or the equilibrium composition, equation A.12 that controls. A final check can be made and this is when k2 = 0, there is no back reaction, equation A.19 goes to equation A.8. The solution of this series of equations is given in many physical chemistry textbooks, but they do not give the details of the integration process and just show it.
The result is certainly not obvious, but it can be easily obtained, although it requires some familiarity with ordinary differential equations. For completeness and to satisfy the curiosity of those more familiar with ordinary differential equations, the details of the calculations are given.
Concentration of A
Concentration of B
And the equation for d[B]/dt, equation A.24, suggests a particular solution, yp, of the form: yp=C e2 −k t1, where C2 is just another constant of integration.
Concentration of C
Slower Reaction Controls [C]
- Example: Calculating Q and k 0 from Kinetic Reaction
- Example: Atmospheric Pressure in Denver 103 4.5.1.2 Example: Atmospheric Pressure at the International
- Example: The Thickness of Earth’s Atmosphere 104
- INTRODUCTION
- THE ARRHENIUS EQUATION .1 O rigins
- Example: Calculating Q and k 0 from Kinetic Reaction Rate Constants and Temperature
- THE HINDENBURG DISASTER .1 C OmbustiOn Of H ydrOgen
- H ydrOgen and tHe H indenburg
- a m aterials f ailure ?
- CONTROLLED COMBUSTION REACTIONS .1 H ydrOgen –O xygen C OmbustiOn
- C OmbustiOn s yntHesis
- BAROMETRIC FORMULA .1 d evelOpment
- Example: Atmospheric Pressure in Denver
- Example: The Thickness of Earth’s Atmosphere
- BOLTZMANN DISTRIBUTION
- THE ACTIVATED STATE .1 a CtivatiOn e nergy
- r Ole Of a C atalyst
- SUMMARY
- Temperature and Speeds of Gas Molecules 113 5.2.2 Maxwell–Boltzmann Speed Distribution 114
- Flux of Gas Atoms Impinging on a Surface 117 5.2.5 Need for High Vacuum in Surface Studies 118
- Gas–Solid Reactions in Materials Processing 122
- INTRODUCTION
- Overview of Various Deposition Processes 124
- Carbon–Carbon Composites on the Shuttle 135
- General Observations about Reaction-Controlled Growth 138
- Reaction Rate Constant and Accommodation Coefficient 139 .1 Example: Condensation of Water Vapor to Ice 140
- GAS KINETICS
- T emperaTure and S peedS of G aS m oleculeS
- m axwell –B olTzmann S peed d iSTriBuTion
- m ean m olecular S peed
- f lux of G aS a TomS i mpinGinG on a S urface
- n eed for H iGH V acuum in S urface S TudieS
- l anGmuir a dSorpTion i SoTHerm
- GAS–SOLID CORROSION REACTIONS
- GAS–SOLID REACTIONS IN MATERIALS PROCESSING .1 T iTanium m eTal and T i o 2 p iGmenTS
- S iemenS p roceSS
- o pTical f iBerS
- H aloGen B ulBS
- CHEMICAL VAPOR DEPOSITION OF SILICON .1 i nTroducTion
- Chemical Vapor Deposition
- Epitaxy
- Sputtering
- T He cVd of S ilicon
- d epoSiTion of S ilicon from S i Hc l 3
- ACTIVE GAS CORROSION OF Si IN HCl AND H 2
- HALOGEN BULBS
- CARBON–CARBON COMPOSITES .1 i nTroducTion
- c arBon –c arBon c ompoSiTeS on THe S HuTTle
- f aBricaTion of c arBon f iBerS
- GENERAL OBSERVATIONS ABOUT REACTION-CONTROLLED GROWTH
- r eacTion r aTe c onSTanT and a ccommodaTion c oefficienT
- SUMMARY
A is the constant, the pre-exponential term, whose units depend on the order of the reaction. Was it a spark caused by a buildup of electrostatic charge on the airship's cladding? The higher the value of the reaction rate constant, the more likely the combustion will be rapid and adiabatic.
However, producing them in ship exhaust is probably not a cost-effective way to make materials. Finally, most of the Pt sites on the surface are covered with single H or D atoms. On the influence of carbonic acid in the air on the temperature of the earth.
In most cases the interest is in the average speed of the atoms and not in their RMS speed. Only 1/6 of the atoms per unit volume, η, move in the z-direction at any given time and only those hit the surface. If there is no pressure of the compound in the gas phase, dichlorobenzene will sublime.
Of practical importance is the sublimation rate in terms of the thickness sublimated in a given time. The transport of the gaseous reactants to and the products away from the surface are usually gaseous diffusion processes. The product of the two factors leads to a maximum in the growth rate at a temperature below the equilibrium temperature.
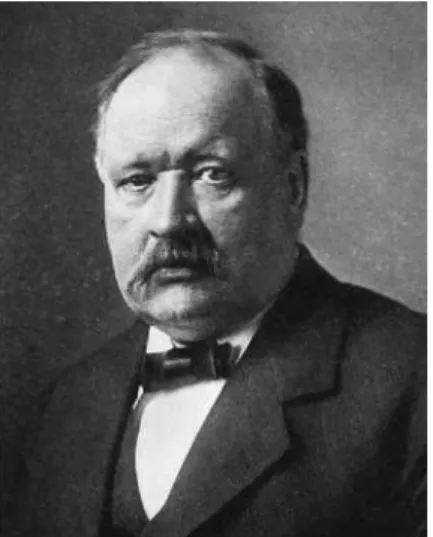
Now the fraction of atoms moving along the axis of this tilted cylinder must be considered. It is assumed that atoms can move in any direction, so the probability that they will cross the surface of a sphere is equally likely in any angular direction. So that the fraction of atoms in any direction at angles θ and φ is the small area of the sphere.
The total area of the sphere is 4πr2 and therefore the fraction of the atoms moving in the direction of θ and φ is .
Thermodynamics of Surfaces and Interfaces and Some Consequences 153
- Anisotropy of Surface Tension and the Shape of Crystals 159
- INTRODUCTION
- Ostwald Ripening by Surface Reaction Control 166 6.8 Freezing Point Depression of Small Particles 169
- Example: Liquid FeO at 1369°C 176
- Example: Water with Sodium Dodecylsulfate,
- Surface Segregation in Other Materials 179
- SURFACE ENERGY, TENSION, AND STRESS .1 S urface e nergy and S urface T enSion
- S urface S TreSS and S urface T enSion
- SURFACE TENSION IN MATERIALS .1 i mporTance
- V alueS of S urface T enSion
- S urface e nergieS and B roken B ondS
- a niSoTropy of S urface T enSion and The S hape of c rySTalS
- SURFACE TENSION AND SURFACE CURVATURE
- CURVATURE AND VAPOR PRESSURE .1 T he m odel
- Example: Water Drop at 25 ° C
- CURVATURE AND SOLUBILITY
- OSTWALD RIPENING BY SURFACE REACTION CONTROL
- FREEZING POINT DEPRESSION OF SMALL PARTICLES .1 a pplicaTion of The c lauSiuS –c lapeyron r elaTion
- SPECIFIC SURFACE AREA
- WETTING
- INTERFACIAL ENERGIES AND MICROSTRUCTURE
- INTERFACIAL ENERGIES AND SURFACE SHAPE
- CAPILLARY RISE .1 T he m odel
Adamson is not the only one concerned about the use of the term surface tension. The number of broken bonds times about half the single bond energy gives a rough estimate of the surface tension. Only in this case is this representation used to calculate the anisotropy of the surface tension.
The length of a line from the center of the graph of γ(θ) to a plane tangent to γ(θ) is proportional to the surface energy of that plane. Note that the surface energy of the crystal face in the drawing plane is not considered in this two-dimensional construction. In the case of the right circular cylinder in Figure 6.8b, r2 = ∞ because the side of the cylinder is straight, so ΔP = γ/r1.
As shown earlier, the rate of growth - or shrinkage - of the particles can be obtained from the molar flux on - or from - the surface of the particles. If all the particles change size, the distribution and mean size of the particles are also changing. Determining the particle size distribution produced by roughening makes model development much more complex.
In this case, the surface of the material is polished in the same way as when preparing the surface for chemical etching.
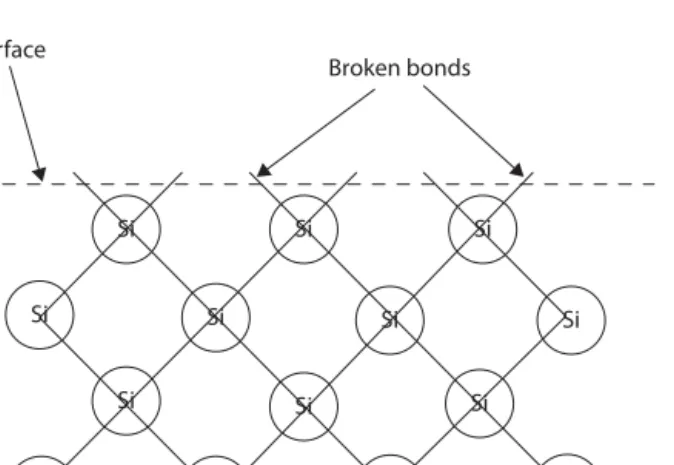