Abstract A qualitative review is given of the theoretical problems and opportunities that arise when one tries to understand what happens in relativistic heavy ion collisions. Of the many different theoretical models invented and applied to the development of hadron physics, there is one - the 'thermodynamic model' [2], which attempts to describe only those aspects of high-energy particle collisions which are extremely similar to the main ones. of relativistic heavy ion collisions, that is, the many-body aspects with an intimate mixture of coherent collective and incoherent stochastic motions. It is therefore not surprising that some ideas of the thermodynamic model have been independently rediscovered by people dealing with relativistic heavy ion collisions.
Some simplistic assumptions can be grossly wrong when extended to the relativistic collision of heavy ions. In high-energy hadron collisions, the common motions have only components in the direction of the collision axis. All the kinetic energy of the incoming particles, which disappears by slowing down the hadronic matter, is adiabatically and locally converted into excitation energy (heat).
Figure 26.1 is a picture of the distribution at the moment of impact, while Fig. 26.2 shows a time evolution, on the left in a model [13] and on the right in a simplified hydrodynamic calculation [14]. Therefore, the excitation energy of a fireball must be a function of initial and current velocity. It has the advantage of being physically visible, since .1/=.01/ is the ratio of the actual kinetic energy density to the initial density of a decelerated volume element.
Also, it will become distorted and its matter content will be mixed through mutual interpenetration with that of the colliding partner.
![Fig. 26.1 Velocity distribution in a collision at the moment of impact, interpreted as continuous (on left) or as a probability distribution of fireballs (on right), from [12]](https://thumb-ap.123doks.com/thumbv2/1libvncom/10206278.0/6.659.85.577.91.225/velocity-distribution-collision-interpreted-continuous-probability-distribution-fireballs.webp)
Statistical Bootstrap Thermodynamics
Equation (26.19) states that the partition function is the Laplace transform of the density of states. What finite number of states, included in the integral of Eq. 26.26) for lnZ cannot do is generate a singularity of the partition function, in other words generate a phase transition. In fact, only the complete spectrum can represent the full interaction; it corresponds to the eigenphase representation of the S matrix [30].
Therefore the function.m/is at the same time:. the density of states of a composite system confined to its natural volume, and the mass spectrum of components of such a system,. and generates the interaction it generates. In fact, the arguments are much more subtle, and the equation for.m/ is not as simple as Eq. 26.28), but the conclusion remains the same: it is of exponential type. We insert the exponential mass spectrum of Eq. 26.26) for lnZ, combine the two nonexponential functions.
0 h.m;T/em=TOem=Tdm; (26.30) where it is obvious that this integral exists for T < T0 and has a singularity at T D T0 whose character depends on h.m;T/and does not interest us at present. Therefore, the asymptotic part of the mass spectrum for any fixed baryon number b must be the same and equal to the pionic one. 0 hN.m;T/em=T0em=Tdm: (26.33) The factor em=T is proportional to the probability of creating a mass m at temperatureT.
In reality, people work differently and lnZN has a slightly different shape, the critical curve in the.T; /plane is not trivial to compute [35] and resembles the curve in Figure 26-7 rather than a straight line. 26.37) is confirmed not only by low-energy nuclear physics, but also by pocket models [3]2 and by the statistical bootstrap model, as can be seen as follows: In the rest frame this reduces to (26.37) and is therefore the unique generalization of (26.37) and of the corresponding low-energy nuclear property.
Coming back to the partition function of a macroscopic system, we now introduce the notion of the available volume [35]. whereVis the externally given volume that encloses the system in a heat bath [for such a system, Eq. 26.38) is obviously not true; it only applies to each of its constituents]. The problems seem great since Zptis is equal to toeZ1, the one-particle function [see Eq. 26.27)], and yet we will introduce a quantity that depends on all momenta and even fluctuates. Therefore we can now consider hVi as a variable that can be prescribed and we can therefore Eq. 26.38) without considering the implicit dependence on hVionhEi:.
This thermodynamics, combined with a good description of the collective motions, should then provide a model for the collision of relativistic heavy ions:. 26.55) While the local bootstrap thermodynamics is known, we still know little about the collective motions, which themselves again depend on the local thermodynamics.
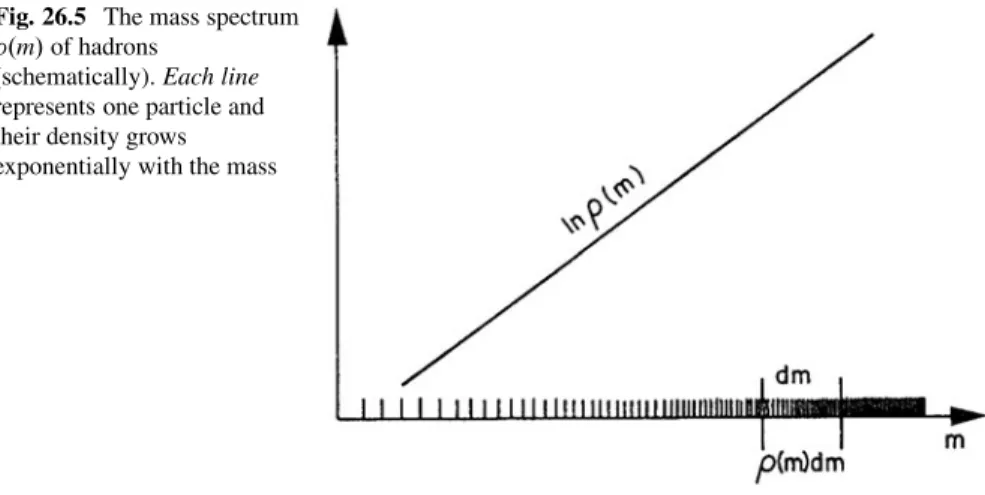
Is There Equilibrium in the Relativistic Heavy Ion Collision?
This thermodynamics, combined with a good description of the collective motions, should then provide a model for the relativistic heavy ion collision:. 26.55) While the local bootstrap thermodynamics are known, we still know little about the collective motions, which themselves again depend on the local thermodynamics. The large transverse momenta can be understood to be due to pre-hadronization processes that take place in the quark-gluon phase and emit a certain energetic quark or gluon before crossing the critical curve. In any case, models along the lines described in the previous sections have been used topA and AA collisions.
0/, where 0 was adjusted to represent a single moving fireball with thermodynamics restricted to the lower, explicitly known part of the spectrum. Malfliet [25] derived a collective velocity distribution [in the sense of F./, but taking into account its temporal evolution] from the relativistic Boltzmann equation. The system approaches this value from below or from above depending on the initial valuea.0/, as shown in Fig.26.10.
However, this is only possible if the total volume available to the whole system is large enough to allow the initially created pairs to escape to distances that are large compared to the range of the destruction interaction. Many intermediate situations can occur in the relativistic collision of heavy ions between such a small and a very large volume. Therefore, it is important to know the volume dependence of the equilibrium distributiona.1/, which can vary between exp.2mA=T/and exp.mA=T/[45].
The time required to reach equilibrium depends on the values of ˛ and ˇ, which determine the slopes of the curves in Figure 26.10. Equilibrium is achieved when ja.t/a.1/j2 is the order of natural fluctuations in equilibrium, i.e. Two approaches have been discussed in the literature [41,43]: constant energy and constant entropy expansion.
We therefore advocate the calculation of cooling curves given by (26.65) as a sequence of quasi-equilibrium states [35]. We can visualize this as in Figure 26-11, where we imagine the dividing walls are coming down one after the other, each time waiting for a new equilibrium to be reached. It is clear that the process depicted in Figure 26-11 is only an approximation of reality, since equilibrium is not reached after each decay step.
![Fig. 26.9 Transverse momentum distribution of 0 at ISR energies [39]. The curves (hand drawn by the present author) can be well approximated by a superposition of three exponentials](https://thumb-ap.123doks.com/thumbv2/1libvncom/10206278.0/27.659.167.501.93.489/transverse-momentum-distribution-energies-present-approximated-superposition-exponentials.webp)
Conclusions
This state will be the one recorded in our particle detectors, but the particles emitted throughout the process will be superimposed on it [35]. But at first it will be a much better approximation than that obtained by assuming constant entropy. For these measurements several events must be taken; pushing for central collisions or selecting events according to any specific criteria would distort the picture.
Such an asymmetry should arise from conservation of angular momentum [46] and be large in peripheral, small in central collisions. While the first type of azimuthal asymmetry would still maintain symmetry with respect to reflection on the collision axis, even it can be destroyed by the “hot spot” mechanism [ 29 ], leading to a right-left asymmetry. Any process originating from that phase and surviving the return to the hadron phase would leave traces of the kind mentioned.
Acknowledgments Much of what has been said here is due to a long and fruitful collaboration with J. Open Access This book is distributed under the terms of the Creative Commons Attribution Non-Commercial License which permits any non-commercial use, distribution and reproduction in any medium, provided the original author(s) and sources are credited.
Frautschi, Ericson fluctuations and the new Argonne data on N scattering, in Hadron Matter at Extreme Energy Density, eds. Cerulus, a fluid droplet model for high energy elastic and inelastic scattering, in Proceedings of the Colloquium on High Multiplicity Hadronic Interactions (Ecole Polytech., Paris III Session, 1970).