List of Tables
Unsteady case
Steady case
Physical Basis
- Magnetohydrodynamics
- The Dimensionless Parameters
- Suction and Injunction
- Free and Forced Convection
- MHD Boundary Layer
- Heat and Mass Transfer
- Rotation
- Thermal and Mass Diffusions
When the Reynolds number of the system is small, the viscous force dominates and the effect of viscosity is important throughout the velocity field. This is the ratio of the viscous diffusivity to the chemical molecular diffusivity and is defined as. Various means have been proposed to prevent the separation of the boundary layer currents; suction and injection are two of them.
The thickness of the magnetic boundary layer is very large and the induced magnetic field is negligible. An exact analysis was made by Soundalgekar et al. 1979) of the effects of mass transfer and the free convective currents on the MHD Stokes (Rayleigh) problem for the flow of an electrically conductive, incompressible viscous fluid past an impulsively started vertical plate under the influence of a transversely applied magnetic field. Georgantopolous and Nanousis (1980) investigated the effects of mass transfer on the free convection current of an electrically conductive viscous fluid (e.g., from a stellar atmosphere) along an impulsively initiated infinite vertical boundary surface (e.g., from a star) in the presence of transverse magnetic material. field.
It was observed that the destabilizing effect of the heat source parameter is more prominent for small values of the magnetic field parameter and the Taylor number. An exact solution of the temperature profile in the MHD flow in a rotating straight channel is derived by Bhat (1982).
The Basic Governing Equations
When an electrically conducting fluid moves through a magnetic field of strength H (B . where B is the magnetic field), equation (2.15) becomes the MHD equation in the following form. Joule's heating expression, where J 2 = o' 2 i(vH. —wH)2 +cr' 2 p(wH —uH)2 +o-' 2 p(uH —vH)2, a' is the electrical conductivity, C is the species concentration variable, 0 denotes the dissipation function, which includes the viscous stress, and represents the rate at which energy is dissipated per unit volume due to viscosity action. In fact, energy is dissipated in a viscous fluid during motion due to internal friction and for an incompressible fluid .. 2.20), which is always positive, since all terms are quadratic, where p is the viscosity coefficient.
Ohm's general law in the absence of an electric field (Maye, 1958) is of the form. It is also assumed that the current is in the x direction, which is carried along the plate in an upward direction and the y axis is perpendicular to it. We have assumed that the magnetic Reynolds number of the current is considered small enough so that the induced magnetic field is negligible compared to the applied number (Pal, 1962), therefore the magnetic field has the form B = (0, B0, 0) and the magnetic lines of force are fixed with respect to the fluid.
The x-component momentum equation reduces to the boundary layer equation if the only contribution to the body force is gravity, the body force per unit mass is F = —pg, where g is the local acceleration due to gravity, further no body force exists in the direction. Therefore, the x - component of the momentum equation becomes ap = —pg, where p is the density of the surrounding fluid at temperature T.
Since the viscous boundary layer and the thermal boundary layer are both small in this case, let 8 be the thickness of the thermal boundary layer and let e <<1 be the order of magnitude of 8, i.e. The detailed descriptions of the present problem are similar to those of case-I and are therefore not repeated here for the sake of brevity. Let the viscosity of the liquid be small and 8 be the thickness of the boundary layer.
Since the viscous boundary layer and the thickness of the thermal boundary layer are the same in this case, let S be the thickness of the thermal boundary layer and let s <<1 be the order of 8, i.e. 0(8) = s. Since the viscosity is very small, and thus the small order terms are neglected, we have equations.
The Calculation Technique 3.1. The Shooting Method
Using this type of numerical technique described above, a computer program will be set up for solving the basic nonlinear differential equations of our problem, where the integration technique will be adopted as the six-order Runge-Kutla method of integration. Based on the integrations performed with the above numerical technique, the obtained results will be presented in the relevant section.
Viscous dissipation and Joule heating effects on unsteady MHD combined heat and mass transfer flow through a
Introduction
Viscous dissipation and Joule heating effects on an unsteady MHD combined flow of heat and mass transfer through a. Therefore, our objective is to investigate the unsteady MHD combined flow of heat and mass transfer through a porous medium past an infinite vertical porous plate with viscous dissipation and the effects of Joule heating in a rotating system.
The Governing Equations
Mathematical Formulations
Since a is both a scale factor and a similarity parameter, any value of 'c' in (4.43) would not change the nature of the solution, except that the scale would be different. The next section discusses the skin friction, Nusselt number, and Sherwood number of the problem.
Skin-friction coefficients, Nusselt number and Sherwood number
Numerical Solutions and Calculation Procedure
The expansion of the Nachtsheim-Swigert iteration shell to the above system of differential equations is straightforward.
Results and Discussion
It can be seen from Fig. 4.5 that the primary speed increases with the increase of Sore. We see from this figure that the secondary velocity field increases with the increase of Magnetic parameter M. It is seen from this figure that rotation parameter has a greater decreasing effect on the secondary velocity with the increase of R.
This figure shows that the temperature field decreases with the increase of the suction parameter v0. From this figure it can be seen that the temperature field decreases with the increase of the Soret number S0. We see from these figures (figures) that the temperature field increasing with the increase of D, E and D maintains a uniform increasing behavior.
From this figure we can see that the temperature field has a large decreasing effect with increasing Prandtl number P. From this figure it can be seen that the concentration field has a large decreasing effect with increasing Schmidt number S. From this figure we can see that with increasing permeability parameter K it is negligibly increasing effect on concentration field.
From table 4.4 we see that the skin friction components r., rz and the Nusselt number N decrease, while the Sherwood number S, increases with the increase of the Eckert number E. From table 4.5 we see that the skin friction components r, r and Sherwood- the number S, increase, while the Nusselt number Nu decreases with the increase of the Schmidt number S.
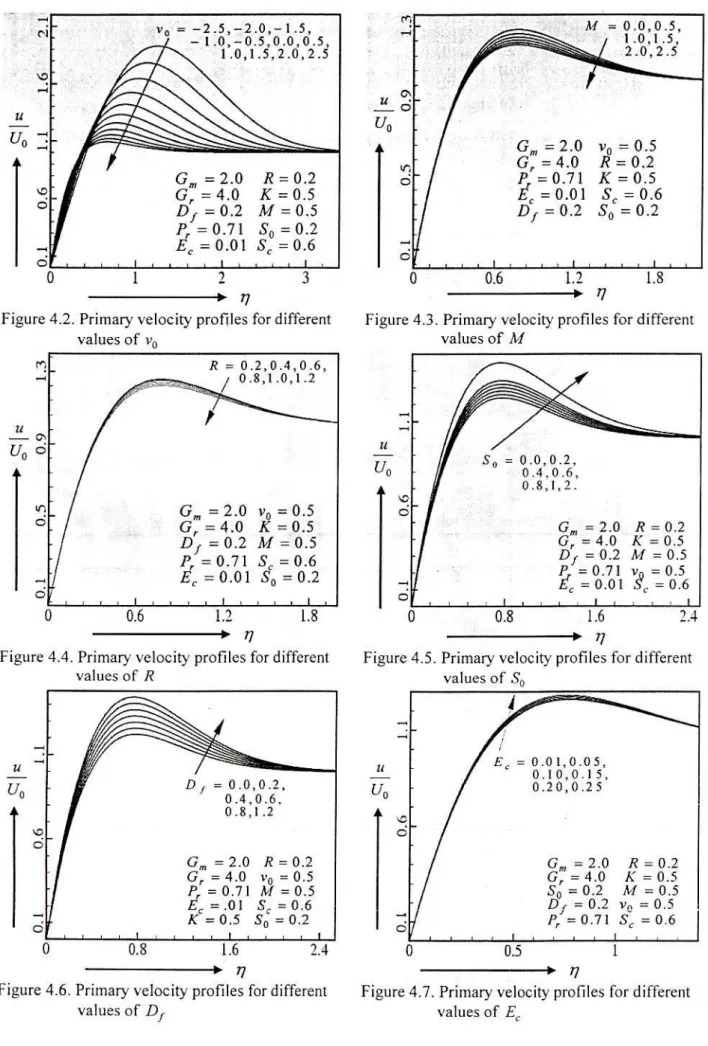
Chapter 5
Viscous dissipation and Joule heating effects on Steady MHD combined heat and mass transfer flow through a
Introduction
The Governing Equations
Mathematical Formulations
The next section deals with the skin friction coefficients, Nusselt number and Sherwood number for the problem.
Skin-friction coefficients, Nusselt number and Sherwood number
Numerical Solution
Results and Discussion
The variation of the primary velocity for different values of rotation parameter R is shown in Fig. It is seen that the Rotation parameter R has a slight decreasing effect on the primary velocity. 5.5-5.7 the variations of the primary velocity for different values of Soret number S0 , Dufour number D1 and the Eckert number E are shown respectively.
From these figures it is seen that the primary velocity increases uniformly with the increase of Soret number 5,., , Dufour number D and Eckert number E the variations of the primary velocities for different values of Prandtl number F,., Schmidt number S, Permeability parameter K are shown respectively. 5.13-5.16 the variations of the secondary speed for different values of rotation parameter R, Soret number S0, Dufour number D and Eckert number E are shown respectively. From these figures, it is seen that the secondary velocity decreases with the increase of rotation parameter R, Soret number So, Dufour number D and Eckert number E.
5.17-5.19 shows the changes in the secondary speeds for different values of the Prandtl number, the Schmidt number S, and the permeability parameter K. These figures show that large increasing effects occur on the secondary speed with an increase in the Prandtl number P, the Schmidt number S, and the permeability parameter K. It can be seen from these figures that the temperature field increases uniformly with increasing Dufour number D and Eckert number Ec The temperature field is shown in fig.
From these figures, it can be seen that the concentration increases smoothly as the suction parameter f increases, while the magnetic parameter M has a small increasing effect on the concentration with the increase of M. 5.35-37, the concentration profiles for different values of Prandtl number P , Schmidt number S and permeability parameter K are shown respectively. From Table 5.3 we see that the skin friction components r and the Nusselt number Nu decrease, while the Sherwood number S1 increases with the increase of Sore.
It can also be seen from this table that the skin friction components r, T: and Nusselt number N 1 increase while Sherwood number S decreases with increasing Prandtl number P.