SEM images of (a) commercial and (b) colloid-synthesized ZnS powders after heat treatment at 1000℃ for 4 h in flow. XRD patterns of CLS ceramic samples sintered via FAST at. different temperatures for 10 min under a uniaxial pressure of XRD patterns of thermally sintered 0.5ZnS-0.5CLS ceramics. pressing at different temperatures, under a uniaxial pressure of.
INTRODUCTION
Infrared Optical Ceramics
Background on Infrared Optical Ceramics
Therefore, a single crystal has few sources of scattering loss, such as pores, defects, and grain boundaries, which allows single crystals to have comparable transparency to glass in the visible and infrared wavelength ranges. Thus, single crystals are attractive candidates for optically transparent materials, especially for applications in harsh environments. In addition, all these techniques require very complex devices, making the growth of single crystals expensive and time-consuming.
Research and Development of Infrared Optical Ceramics
A high packing density can be helpful for further densification and pore removal during the sintering process of infrared optical ceramics. Pressure-assisted sintering techniques, including hot press and field-assisted sintering techniques, are widely used in the sintering of infrared optical ceramics. Annealing is used for restoring transparency by eliminating vacancies within infrared optical ceramics.
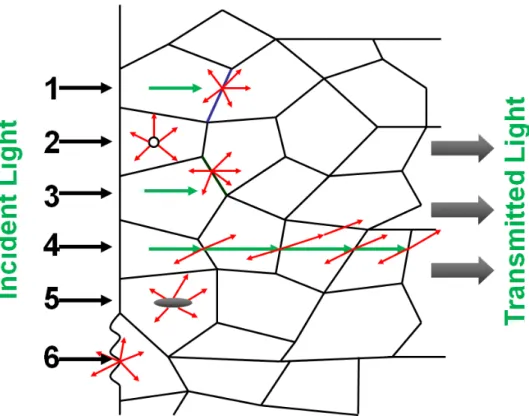
Sulfide-Based Infrared Optical Ceramics
ZnS Infrared Optical Ceramics
Two well-characterized crystal structures of ZnS (ZnS, in mineral form at ambient pressure, experiences a phase transition at 1020 ºC from the cubic sphalerite phase to the hexagonal wurtzite phase). This work led to the commercialization of ZnS infrared optical ceramics, called IRTRAN® 2.51. It was claimed that the ZnS polycrystalline optical ceramics with high relative density (higher than 99%) were produced by hot pressing of ZnS powder, with the particle size of less than 5 µm. Isogai et al.134 recently reported the fabrication of ZnS infrared optical ceramics by optimizing sintering conditions during SPS.
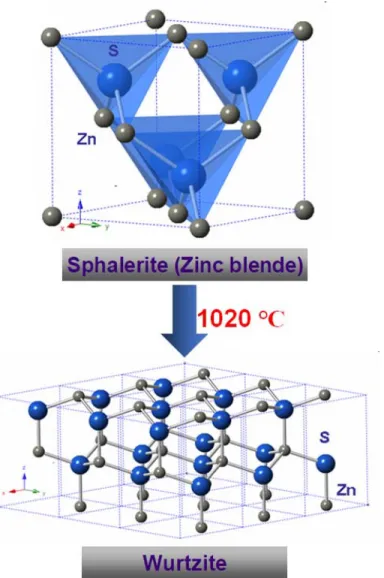
CaLa 2 S 4 Infrared Optical Ceramics
At the same time, researchers in the United States also conducted massive processing studies on CaLa2S4 infrared optical ceramics. The researchers further investigated the correlation between chemical compositions and optical properties of the CaLa2S4. CaLa2S4-La2S3) infrared optical ceramic. They applied two sintering procedures (hot pressing in vacuum or H2S; sintering in H2S followed by HIPing in argon) to consolidate the CaLa2S4 infrared optical ceramics.
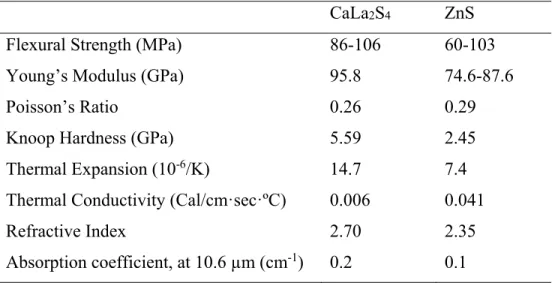
Sulfide-Based Composite Infrared Optical Ceramics
The infrared transmittance of the ZnS diamond ceramic significantly depended on the quality of the diamond dispersion, which was closely correlated with the green mode processing conditions. The EMA model was used to explain the absorption in the two-phase media, based on the microstructure observation, which showed that grains of the two phases were similarly connected in the composite ceramic.208. Fujii et al.209 reported that the hot pressed ZnS diamond composite ceramic with increased hardness, fracture strength and Young's modulus could improve the rain erosion durability of the infrared optical ZnS ceramic.
Goals and Organization of the Thesis
PHASE TRANSITION BETWEEN SPHALERITE AND
Introduction
Scientists have conducted remarkable studies on the phase transition in ZnS optical ceramics processed by various routes. Nevertheless, few studies have been conducted focusing on the effect of initial particle size and hot pressing pressure on the phase transition behavior of ZnS optical ceramics. The effects of initial particle size and sintering pressure on the phase transition between 3C-sphalerite and 2H-wurtzite transition of ZnS were studied in detail.
Experimental Procedures
The phase composition of the ZnS powders and bulk ceramics was determined by means of XRD (D2 PHASER, Bruker) equipped with CuKα radiation (30 kV, 10 mA), with the microstructural features of the powders characterized with SEM (Quanta 200, FEI ). A Tristar II 3020 system (Micromeritics) was used to measure the surface area of the ZnS powders using the BET method. All the EBSD data were collected from the polished surfaces of the ZnS ceramic samples.
Results and Discussion
SEM images of the (a) commercial ZnS powder and (b) colloid-synthesized ZnS powder used in this study. SEM images of the (a) commercial and (b) colloid-synthesized ZnS powders after heat treatment at 1000 ℃ for 4 h in flowing argon. XRD patterns of the commercial ZnS powder before and after heat treatment at 1000 ℃ for 4 h in flowing argon.
XRD patterns of the colloid-synthesized ZnS powder before and after heat treatment at 1000 ℃ for 4 hours in flowing argon. The XRD patterns of the ZnS ceramic sintered via both pressureless sintering and hot pressing using the two heat-treated powders are shown in Figures 15 and 16. via hot pressing under 50 MPa.
XRD patterns of the heat-treated colloid-synthesized ZnS powder, and the resulting ceramic sintered without pressure and by hot pressing under 50 MPa. 0001} pole figures of the wurtzite phases within the hot-pressed ZnS ceramics sintered under different pressures using different powders: (a) under 50 MPa using heat-treated commercial powder; (b) below 50 MPa using heat-treated synthesized powder; and (c) below 10 MPa using heat-treated synthesized powder. The overall spectra of the two hot-pressed ZnS ceramics are similar, indicating similar vibrational bonds in the two samples.
Infrared transmission spectra and photographs of the ZnS ceramics sintered via hot pressing at 1000 ℃ for 2 hours under 50 MPa, using heat-treated commercial and colloid synthesized powders.
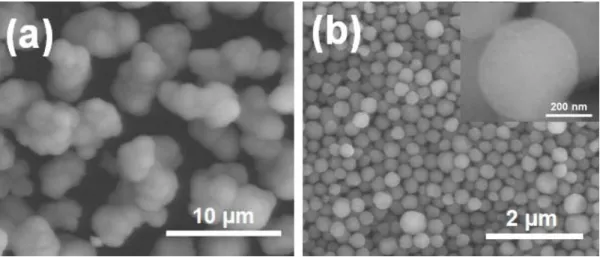
Conclusions
HOT-PRESSED Cr 2+ DOPED ZnS INFRARED TRANSPARENT
- Synthesis with High-Temperature Sulfurization
- Synthesis without High-Temperature Sulfurization
CONSOLIDATION OF CaLa 2 S 4 INFRARED OPTICAL
SEM images of synthesized precursors obtained by different wet chemistry routes: (a) coprecipitation, (b) carbonate precipitation, and (c) combustion. XRD patterns of CLS precursors sulfurized at 1000 ºC in a CS2/Ar atmosphere for 8 hours. The presence of oxygen can be attributed to oxygen attached to the pores and surfaces of the sulfurized CLS precursors.
SEM images of CLS precursors synthesized by wet chemical precipitation using (a) TAA, (b) thiourea, (c) (NH4)2S, and (d) Na2S as sulfur sources. XRD patterns of CLS precursors after thermal decomposition in an Ar atmosphere at 1000 °C for 5 h. XRD pattern of the synthesized CLS powder after thermal decomposition at 1000 °C for 5 h in liquid argon.
Reaction scheme for the synthesis of CLS by the wet chemistry method followed by thermal decomposition. The oxygen peak is due to oxygen attached to the pores and surface of the CLS powder. Morphological and microstructural characteristics of CLS powders and ceramics were investigated by SEM (Quanta 200, FEI).
In this case, average grain sizes of the CLS ceramics are used for plotting to determine the m value. Further investigation of the infrared optical transmittance of the CLS ceramics sintered via FAST was performed by FTIR measurements and analysis. The Vickers hardnesses of the CLS ceramics sintered via FAST are shown in Table IV.
PRESSURE-ASSISTED CONSOLIDATION OF ZnS-CaLa 2 S 4
The theoretical density of the composite ceramic was calculated based on the phase composition of the materials. SEM images of the as-synthesized ZnS powders (a), commercial CLS powders (b), heat-treated ZnS powders (c), and ball-milled 0.5ZnS- 0.5CLS powders (d). XRD patterns of the as-synthesized ZnS powders, heat-treated ZnS powders, commercial CLS powders, and ball-milled 0.5ZnS-0.5CLS.
Temperature dependence of actual piston distance and speed measured during field-assisted sintering of 0.5ZnS-0.5CLS composite ceramics at 1050 ºC, under uniaxial pressure of 100 MPa for 20 minutes. A detailed grain size analysis was performed to investigate the grain growth of 0.5ZnS-0.5CLS ceramics sintered in the temperature range of 800–1050 ºC. Grain size distribution of ZnS and CLS in 0.5ZnS-0.5CLS ceramics sintered by FAST at different temperatures under a.
XRD patterns of 0.5ZnS-0.5CLS ceramics sintered via FAST at different temperatures, under a uniaxial pressure of 100 MPa for 20 minutes. The TEM investigation provides detailed information on the sphalerite-to-wurtzite transition of the ZnS component in the composite ceramics. It is also established that the hardness of ceramics depends on the sintering temperature.
The XRD patterns of the 0.5ZnS-0.5CLS ceramics sintered at different temperatures are shown in Figure 69.
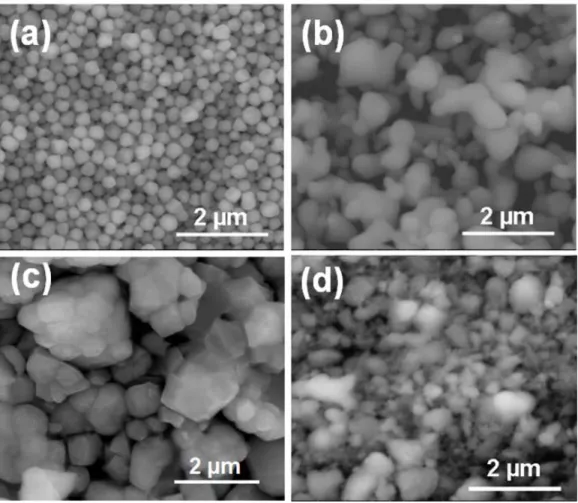
CONCLUSIONS
Band gap enhancement is observed due to the nanometric nature of the crystallites that make up the powder agglomerates. For the ceramics sintered via field-assisted sintering at 850-1100 ºC under 50 MPa, densification in the 850-950 ºC temperature range has been determined to be controlled by a mixture of lattice or grain boundary diffusion and grain boundary sliding. It has been revealed that grain boundary diffusion is the main mechanism controlling grain growth between 950 ºC and 1100 ºC.
It is proposed that grain growth is controlled by grain boundary diffusion, with activation energies of 57.97 kJ/mol and 150.22 kJ/mol, for samples sintered via FAST at 850-1100 ºC and hot pressing at 950 -1200 ºC, respectively. . It is observed that the hardness of CaLa2S4 ceramics first increases with increasing sintering temperature due to densification, and then decreases at higher sintering temperatures due to significant grain growth. Furthermore, an investigation has been carried out on the sintering behavior, phase transition and hardness of ZnS-CaLa2S4 composite ceramics by pressure sintering of laboratory-processed ZnS nanopowders mixed with commercial CaLa2S4 powders by high-energy ball milling (50%: 50% in volume ratio).
For ceramics sintered via field-assisted sintering at 800-1050 ºC under 100 MPa, it has been found that grain growth of ZnS and CaLa2S4 components is controlled by grain boundary diffusion, in the sintering temperature range of 800-1000 ºC. . XRD analysis was used to determine that the pressure-assisted consolidated composite ceramic consists of ZnS sphalerite, ZnS wurtzite, and CaLa2S4 structured with thorium phosphate, with the presence of wurtzite indicating the early sphalerite-wurtzite phase transition. TEM investigations further suggest that the phase transition of ZnS is accompanied by the formation of stacking faults and twins.
As sintering temperature increases, it is indicated that the hardness of the ZnS-CaLa2S4 composite ceramics first increases due to densification improvement, and then decreases due to the onset of excessive grain growth and effects of thermal decomposition.
FUTURE WORK
Harris, “Development of Hot-Pressed and Chemical Vapor Deposited Zinc Sulfide and Zinc Selenide in the United States for Optical Windows”; pp. Yoshida, “Manufacturing and optical properties of high-performance polycrystalline Nd:YAG ceramics for solid-state lasers,” J. Heinz, “Comparisons of state and optical properties of the high-pressure phase of zinc sulfide,” J.
Johnson, "Effect of post-CVD thermal treatments on crystallographic orientation, microstructure, mechanical and optical properties of ZnS ceramics." 34;Microstructural and optical properties of the ZnS ceramics sintered by vacuum hot pressing using hydrothermally synthesized ZnS powders," J. Sanghera, "Manufacturing and Spectroscopy Pr3+ Doped Ceramic Calcium Lanthanum Sulfide for Mid-IR Laser Gain Materials"; pp.
Zhang, “Synthesis and properties of transition metals and rare earth doped ZnS nanoparticles,” Opt. Li, “Improved thermoelectric properties of bismuth sulfide polycrystals prepared by mechanical alloying and spark plasma sintering,” J. Li, “Thermoelectric properties of Sn-S bulk materials prepared by mechanical alloying and spark plasma sintering,” J.