The present work focuses on transient evolution of pulsed laser induced breakdown process in liquid and solid-liquid interface. In the present work, the complete dynamics of laser-induced breakdown in water and metal-water interface is studied.
Laser Induced Breakdown At Target-Liquid Interface 66
Application of Synthesized Nanoparticles 97
Schematic of BDS at target-water interface 29 2.20 Schematic of LIBS at target-water interface 30 2.21 Experimental setup for synthesis of nanoparticles via laser target. one).
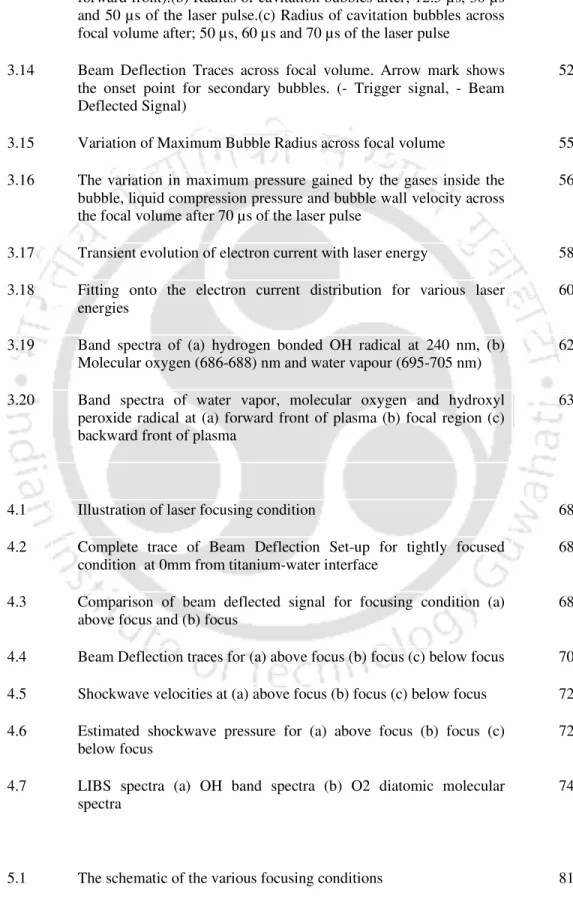
List of Tables
Symbols
Introduction
- Laser Induced Breakdown in Liquids
- Significance and Current status of LIB at liquid/solid-liquid interface
- The present Work
For ns laser-induced breakdown, the plasma formation takes place within the leading edge of the laser pulse. However, very few reports were focused on the complete investigation of the nucleation mechanism of nanoparticles synthesized via laser-induced breakdown at solid-liquid interface [9].
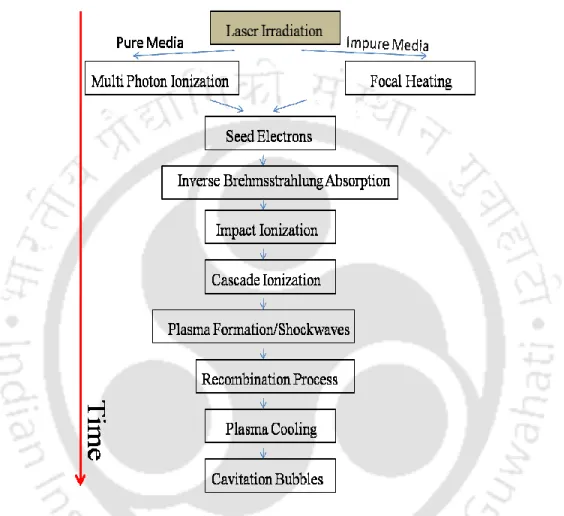
Experimental Details
- Laser Induced Breakdown In Liquids
- Beam Deflection Set-up (BDS)
- Shadowgraphy Technique
- External Electric Probe (EEP)
- Laser Induced Breakdown Spectroscopy (LIBS)
- Laser Induced Breakdown at Target-liquid Interface
- Laser Ablation at Titanium-water interface
- Synthesis and Characterization of Nanoparticles formed at Titanium/Copper-Water Interface
- Application of Nanoparticles
- Antibacterial Activity
- Photocatalytic Activity
A schematic of the experimental setup for studying laser ablation at the titanium-water interface is shown in Figure 2.15. The size of the laser spot on the target for all focusing conditions is shown in Figure 2.22(b).
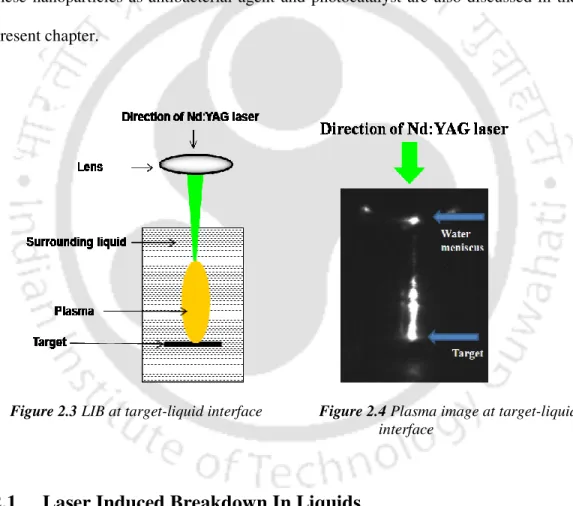
Laser Induced Breakdown in Liquids
- Shockwave and Cavity Dynamics via Beam Deflection Set-up
- Multiple Bubble Interaction via Shadowgraphic Technique
- Transient Evolution of Plasma Charge Carriers via External Electric Probe
- Laser Induced Breakdown Spectroscopy in Water
Therefore, the signals were recorded separately on the extended scale, as shown in Figure 3.2(b), 3.3(b), 3.4(b), respectively. In Figure 3.4(b), the BDS traces show the onset of a second narrow negative dip after shock wave emission. The observed cluster of closely spaced bubbles along the incident laser direction for different time intervals with respect to the Nd:YAG laser pulse is shown in Figure 3.11.
The liquid in the interface zone of two approaching bubbles flows out radially and gives an upward pressure to the trailing bubble. The remaining liquid at the interface becomes trapped and compressed and van der Waals forces become effective [86]. The spatial evolution of the bubble radius across the focal length at different time intervals is shown in figure 3.13. The location of the forward and backward front of the cavitation bubbles relative to the focus is marked as 3 mm as shown in Figure 3.13(a).
It can be seen from Figure 3.13 that the spatial evolution of cavitation bubbles across the focal volume shows a damped oscillation. These results are also complemented by BDS traces at different distances from the focal region, as shown in Figure 3.14. The maximum drop in the photodiode signal of 80 mV is observed in the focus (Figure 3.14 (c)), which gradually decreases on both sides of the focus.
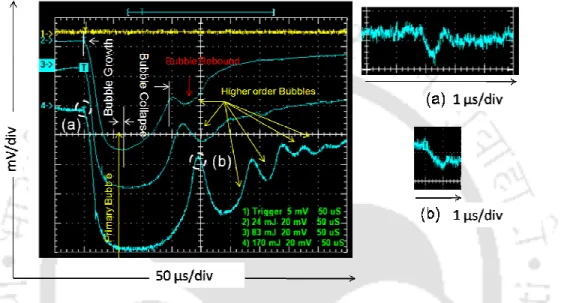
Laser Induced Breakdown at Target-liquid Interface
- Estimation of Pressure at Titanium-Water Interface using BDS
- Estimation of Temperature at Titanium-Water Interface using LIBS
- Implication of Pressure and Temperature on Nucleation of Nanoparticles at Titanium-Water Interface
The BDS setting at the Titanium-Water interface was obtained for different focusing conditions (A, B and C) shown in Figure 4.1 and discussed in detail in Section 2.2. The complete beam deflection traces for the laser focused condition (B, Fig. 4.1) at 0 mm from the target-water interface are shown in Fig. 4.2. The spatial evolution of the shock wave velocity from the titanium-water interface for the condition above the focal point, focal point and below the focal point is shown in Figure 4.5 (a), (b) and (c), respectively.
The estimated shock wave pressure for over focus, focus and under focus condition with the distance from the target is shown in figure 4.6 (a), (b) and (c) respectively. The pressure experienced at the water breakdown region for underfocus condition due to shock waves 2 (figure 4.4 (c)) is 18.3 GPa. LIBS was used for various focusing conditions (figure 4.1) at titanium-water interface for estimation of rotational temperature in the breakdown region.
The plasma-emitted spectrum at the titanium-water interface for the laser-focused condition (B, Figure 4.1) is shown in Figure 4.7. The estimated pressure at the titanium–water interface was found to be 18 GPa for the laser-focused condition (B, Figure 4.1, Section 4.1). Once the TiO2 critical core is formed, it grows under HPHT conditions, but the laser-produced plasma is very transient, so the rapid plasma quenching stops nanoparticle growth down to a few nanometers. The preliminary estimate of the size of the nanoparticles d, is given as [78].
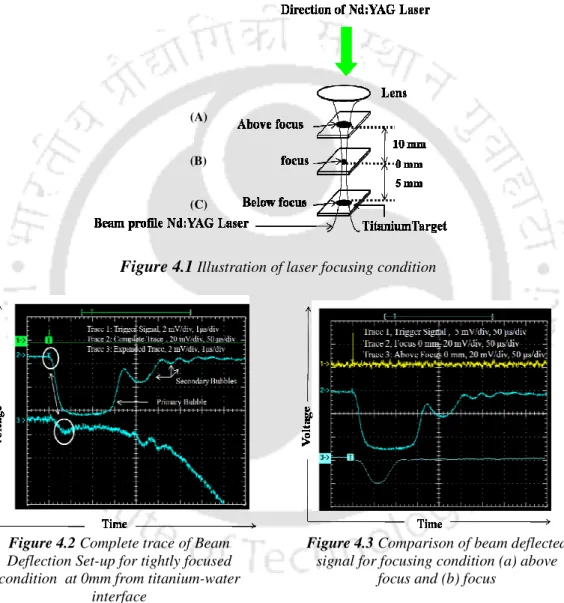
Synthesis of Nanoparticles via LIB at Target- Liquid Interface
- Synthesis of TiO 2 Nanoparticle via Laser Ablation at Titanium- Water Interface
- Effect of Pressure and Fluence on Synthesized TiO 2 Nanoparticles
- Comparision of Estimated and Experimentally derived size of TiO 2
- Synthesis of Copper Oxide Nanoparticle via Laser Ablation at Copper-Water Interface
It is observed from figure 5.6 that the absorption and yield for top focus condition (plot a) is more compared to tightly focused condition (plot b). As discussed in section 1.1., the laser ablation followed by plasma generation occurs only during the leading edge of the pulse. The plasma blocks the trailing edge and absorbs laser energy. The absorption of laser energy by plasma is called shielding effect which is dominant under tight focus.
The TEM images of the synthesized copper oxide nanocolloids under different focusing conditions are shown in Figure 5.10. The structural features of the nanoparticles were revealed using SAD and HRTEM images, depicted in Figures 5.11 and 5.12, respectively. The UV-Vis spectra of the in-situ prepared colloidal solution revealed some interesting features as shown in Figure 5.13.
Synthesized nanocolloids near the laser focus (T1) are deconvolved in the inset of Figure 5.13. The sharp absorption peak at 216 nm is attributed to an interband electron transition of Cu0 near its Fermi level [142,143]. The surface plasmon (SPR) peak of Cu lies in the range 570–590 nm. Raman spectra for colloids synthesized under different focusing conditions at an excitation wavelength of 488 nm are shown in Figure 5.14.
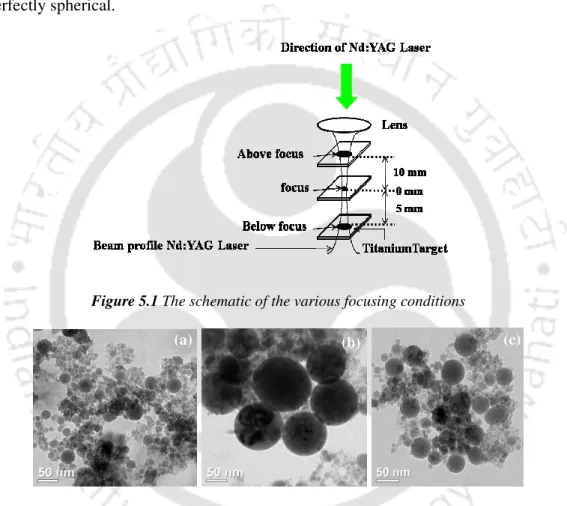
Application of Synthesized Nanoparticles
- Cu@Cu 2 O Nanoparticles as Antibacterial Agent
- Bacterial Inhibition of Gram positive Listeria
- Bacterial Inhibition of Gram negative E. coli
- Bacterial Inhibition of Gramnegative S. paratyphi
- Physical Mechanism behind Antibacterial Properties of Nanoparticles The underlying physical mechanism behind bactericidal effect of Cu@Copper Oxide
- TiO 2 Nanoparticles as Photocatalyst
- Photodegradation of Methylene Blue
- Physical Mechanism behind Photocatalytic Activity
It is clear from figure 6.4 that the intensity of the characteristic Raman scattered radiation of E.coli has drastically reduced after treatment with Cu@Cu2O nanoparticles. This further confirms that the nanoparticles are effective in inhibiting the growth of the bacterial culture. The bacterial limitation of pathogenic S.paratyphi was studied by exposing it to Cu@Cu2O nanoparticles.
Bacterial viability is clearly visible from the bacterial colonies grown on agar plates as shown in figure 6.6. The exponential phase resembles the growth of bacteria as the bacterial cells begin to divide and thereby increase its population. The sudden drop in optical density of figure 6.7 after 10 hours of incubation is attributed to the death phase of bacterial stains.
The ability of nanoparticles to adhere to bacterial cell walls promotes biochemical reactions and causes toxicity to bacterial cells. Further denaturation and solidification of proteins by copper ions can also disrupt the bacterial cell wall [157-158]. In the photodegradation of methylene blue, these surface defect states act as electron scavengers to enhance the photocatalytic activity of a water splitting reaction similar to that shown in Figure 6.10 [162].
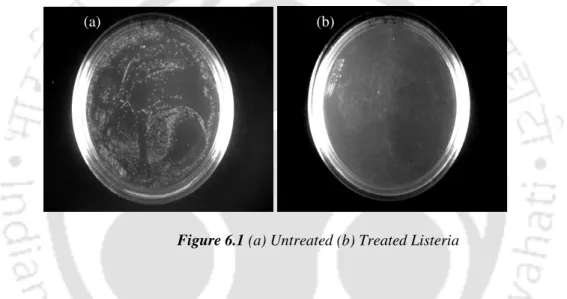
Conclusion
The formation of multiple bubbles in the laser-induced water plasma and the subsequent bubble interaction were studied using the shadowgraphic (SG) technique. Collapse of bubbles at the focal region was observed at 60 µs and formation of liquid jet was visible after 70 µs of the laser pulse. The transient currents due to the formation of plasma were recorded by measuring the voltage drop across 100 resistor connected in series to the grounded electrode of the probe and displayed on the DSO.
Irradiation of high power laser on target leads to plasma formation of the target which interacts with surrounding water molecules to form metal oxide (copper oxide/titanium oxide) nanoparticles. The laser ablation of titanium target immersed in water showed the formation of high temperature rutile phase of TiO2. To understand the nucleation mechanism for the formation of nanoparticles, the pressure of the shock wave (using BDS) and temperature (using LIBS) at the titanium-water interface were measured in the LIB region for various focusing conditions of the Nd:YAG laser.
BDS measurement showed formation of high pressure zone ~ 18 GPa for tightly focused condition on the target, while that of the defocused condition the pressure was 1GPa. For copper oxide nanoparticles, the tightly focused state showed formation of thermodynamically stable copper oxides (CuO) of size 200 nm. The defocused condition showed formation of smaller nanoparticles (<10 nm) with a higher probability of formation of kinetically stable copper oxides (Cu2O).
Future Scope
Liquid Patterning of ArF Immersion Lithography, Research Laboratory Reports, Asahi Glass Co., Ltd., 55, p. 2006). A new technique for the synthesis of silver nanoparticles by laser-liquid interaction, Journal of Materials Science., 33, p. 1999). 1982) Equation of state and electrical conductivity of shocked water and ammonia in the pressure range 100 GPa (1 Mbar), Journal of Chemical Physics., 76, p. 1990).
Validity criteria for local thermodynamic equilibrium in plasma spectroscopy, Physics Review A., 42, p. 1964) Plasma Spectroscopy., New York: McGraw-Hill. The ultraviolet bands of OH Fundamental data, Journal of Quantum Spectroscopy and Radiation Transfer., 2, p. 1991). The growth kinetics of TiO2 nanoparticles from titanium(IV) alkoxide at high water/titanium ratio, Journal of Physical Chemistry B., 107, p 2005).
1999) X-ray diffraction study of Ti-O-C system at high temperature and in a continuous vacuum, Journal of Alloys Compounds., 288, p. 1991). Phase Diagrams of the Elements (University of California Press). 2007) Additives and the Crystallization Process: From Fundamentals to Applications (John Wiley & Sons, Ltd.) p. 1999). Optical absorption and emission studies of 2 MeV Cu-implanted silica glass, Nuclear Instrument and Methods in Physics Research B p 2001).
List of Publications
Size-Induced Structural Modifications in Copper Oxide Nanoparticles Synthesized via Laser Ablation in Liquids, Arpita Nath and Alika Khare (under review). Transient Evolution of Charge Carriers in Laser-Induced Plasmas in Liquids, Arpita Nath and Alika Khare (communicated). Photocatalytic Activity of TiO2 Nanoparticles Synthesized via Laser Ablation in Liquids Arpita Nath and Alika Khare (In preparation).
Estimation of OH and O2 rotational temperature during laser induced collapse at titanium water interface”, Arpita Nath and Alika Khare, MMISLIBS-10, Allahabad, December 2010. Bacterial inhibition by Cu/Cu2O nanocomposites prepared via laser ablation in liquids,”, Arpita Nath Archana Das, Latha Rangan and Alika Khare, Photonics-10, IIT Guwahati, December 2010. Antibacterial activity of copper oxide nanoparticles synthesized via laser ablation in liquids”, Arpita Nath, Archana Das, Latha Rangan and Alika Khare, IISC-10, Jamia Millia Islamia, Delhi, December 2010.
Photocatalytic Activity of TiO2 Nanocolloids Formed by Laser Ablation of Ti in Water," Arpita Nath and Alika Khare, "PEFM 2010 - Physics of Emerging Functional Materials", BARC Mumbai, September 2010. Synthesis of TiO2 Nanoparticles by Laser Ablation at the Titanium-Water Interface", Arpita Nath and Alika Khare, “ICE-2009 – International Conference on Electroceramics”, University of Delhi, December 2009. Spectroscopic Investigations of Laser Induced Decomposition in Water”, Arpita Nath and Alika Khare, Plasma 2008 – National Symposium on Plasma Physics, December 2008, BARC Mumbai.