This thesis presents an origami-based hybrid upper limb control module that has the potential to support workers in the industrial sector. The proposed control module which is pneumatically driven consists of rigid material and soft material to improve power transmission and operating speed while maintaining flexibility. An additive manufacturing method using a heat press and a laser cutting machine was developed to shorten the manufacturing time and easily customize the control module design.
Analytical modeling was established which shows the relationship between origami pattern design parameters and module performance, and the design parameters are optimized to satisfy upper limb support requirements.
List of Tables
Introduction
Recently, wearable systems are in the spotlight in rehabilitation, virtual reality (VR) and industrial fields. As shown in Fig Ⅰ.1.1, the market of portable systems expands drastically every year [43, 44]. Soft wearable systems are easy to adapt to different body sizes due to compliance of materials and structures.
Soft activators that are widely used for soft coating systems can be classified according to their activation principles. Moreover, the length of the cable and the position of the pulley must be set differently depending on the different body sizes of the users. A new fabrication method, an additive manufacturing method was developed to shorten the fabrication time and make it easy to modify the actuator module design.
In Section Ⅲ.1, analytical modeling of origami-based hybrid actuation modules will be proposed. In Section Ⅲ.2, the design optimization process of the upper limb support actuator module will be explained and the optimization result will be discussed.
![Fig Ⅰ.1.2 Issues of conventional wearable systems and the emergence of soft wearable systems [1~4]](https://thumb-ap.123doks.com/thumbv2/123dokinfo/10495944.0/14.892.162.740.201.455/fig-issues-conventional-wearable-systems-emergence-wearable-systems.webp)
Design and Fabrication of the Origami-based Hybrid Actuating Module
Assuming that the same amount of air volume (∆𝑉) is injected into two chambers and the same pressure (𝑝) acts on the inner walls of chambers, the latter one can be extended more in 𝑥 direction than case 1). A new manufacturing method was developed to shorten manufacturing time and to easily change the design of the actuator. In addition, the performance of each actuator varies depending on the skill of the manufacturer.
For the design of the airtight chamber, they provide experimental information on the size and thickness of the materials and the way the interfaces are connected. The manufacturing process allows simple changes to the actuator module design, and rapid manufacturing is also available. They are cut according to the origami pattern with a laser cutting machine and then placed in the middle of the inner wall of the trigger module.
By changing the thickness and hardness of the skeleton, the durability and size of the control module can be manipulated. Skins are the fabric-based materials that cover the outer part of the control module. The stiffness of the control module can be manipulated by changing the thickness of the adhesive.
The melting point of the glue must be lower than that of the skin and the skeleton, because only the glue needs to melt and the skeleton adheres to the skin when heat pressing. Since the stiffness of the drive module depends on the shore hardness and thickness of the glue, the shore hardness must be less than 80A and the thickness no more than 0.2 mm. The TPU [27] was chosen considering the melting point, adhesion, shore hardness and the thickness of the materials.
The skeleton must be durable and light due to the compactness and durability of the drive module. Polycarbonate (PC) was chosen considering the melting point, Young's modulus, impact strength and the density of the materials. The nylon-coated fabric was selected as a sheet taking into account the melting point, air density and thickness of the materials.
First cover the skin and the glue and attach to the top of the cylinder with the heat press (Fig Ⅱ.2.8). Thus, the bending motion can be achieved by blocking the extension of one side of the linear drive module.
![Fig Ⅱ.1.2 Characteristics of origami structures: (a) Programmable curvatures [14], (b) various motions by folding and unfolding processes [17], (c) gadgets for creating foldable origami](https://thumb-ap.123doks.com/thumbv2/123dokinfo/10495944.0/19.892.118.784.351.662/characteristics-structures-programmable-curvatures-unfolding-processes-creating-foldable.webp)
Design Optimization of the Origami-based Hybrid Actuating Module for Upper Limb Support
Based on the average body sizes of adult men in Korea, the reference specification of the actuator module can be calculated. The larger 𝐴, the larger the width of the actuator and the smaller the degree of expansion of the actuator module per module. As 𝑡 increases, the stiffness of the actuator module also increases, which improves the support force that the module can withstand.
Depending on the value of 𝑛2, the shape of the bottom of the control module is changed. The gap between the parts of the skeleton should be 𝑡π to maximize the range of motion of the control module. The quasi-static model is used to formulate the blocked force and range of motion of the control module.
By subtracting 2𝑛2(𝑛1− 1) tetrahedrons on the cylinder, the volume of the actuator module can be calculated. The trapped force of the actuator module can be determined by the energy conservation equation. The maximum bending angle of the actuator module can be formulated with the design parameters (Fig. Ⅲ.1.10).
The blocked force was continuously measured, increasing the extension rate of the drive module from 100% to 280%. However, the larger 𝐴 is, the wider the range of extension rate and the force of the drive module is predictable with the modeling equation. I can find the predictable range with the modeling regardless of the drive module design.
𝐹 is the support force of the drive module and 𝜙 is the bending angle of the drive module. 𝐴, 𝑛1, 𝑛2 and 𝑡 in Fig Ⅲ.2.1 are the optimized design parameters of the drive module which makes the drive module compact. The support force of the drive module must sustain 5 kg, taking into account the weight of the arm and equipment.
It is obvious that 𝑛1 and t are proportional to 𝜙𝑚𝑖𝑛 because 𝑛1 is the modulus number and t is the sheet thickness. The force can be expressed as area × pressure, i.e. the cross-sectional area of the actuator module.
![Fig Ⅲ.1.2 Various shoulder motions [46]](https://thumb-ap.123doks.com/thumbv2/123dokinfo/10495944.0/35.892.134.781.328.884/fig-ⅲ-1-2-various-shoulder-motions-46.webp)
Experimental Verification 4.1 Experimental setup
In order to measure the range of motion and the supporting force of the drive module, an experimental set-up was built using profiles (Fig Ⅳ.1.2). The location of the module can be adjusted with the size of the drive module, and there is a fixed stand at the end of the upper arm part so that some weight can be loaded. In addition, a linear guide is integrated with the upper arm to allow the module to move as the bending angle increases.
Anchoring structures were developed for connecting the activation module to the bodies of users (Fig Ⅳ.1.3). The humerus attachment part and the body attachment part are separately attached to the upper arm and torso of a welding vest. Air pressure is slowly injected into the actuator module and the module lifts the loads.
The support force and the maximum bending angle of the operating module are almost consistent with the values of the simulation. The process of the passive support test without the air pump motor is as follows. Raise the arm and allow air to enter the activation module and close the air hose to seal the air.
Then check that the module can create the sufficient support force and move the arm back and forth to check that the module follows the movement naturally. Like the passive support test, the support force and compliance of the actuation module was verified.
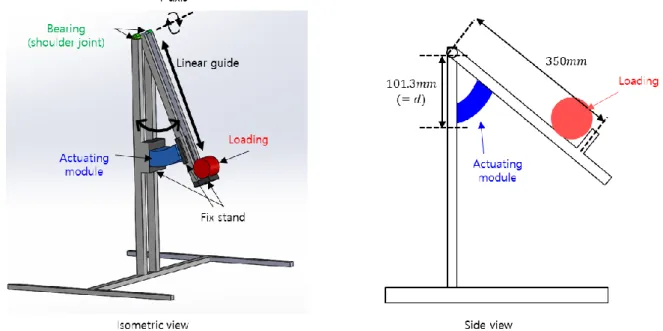
Conclusion and Open Issues
Some checking is required to ensure that the trigger module is not damaged by welding embers. Investigation of other materials is needed to minimize weight and make the system more resistant to other factors such as temperature, humidity, pressure, etc. Analytical modeling is not fully consistent with experimental data due to the compressibility of air and the deformation of rigid plates.
Considering the compressibility of the air, the relationship between 𝑝 and 𝑉 needs to be determined and a new model is needed to predict the performance of the propulsion module.