Fe-Cu-Si ternary composites (FeCuSi). a) Schematic illustration of the structure of FeCuSi and its synthesis process. Si nanolayer-embedded graphite/carbon (SGC) composite. a) Schematic illustration of the manufacturing process of SGC composite.
Introduction
First of all, the necessity of co-utilization of graphite and Si will be discussed from the practical point of view. Furthermore, the critical consideration factors for the development of Graphite/Si Anodes will be deliberately presented with the simple experimental demonstration.
Necessity for co-utilization of graphite and Si in commercial level
Due to the sparse information on the average voltage of the silicon anodes and the difference in the cathode for full-cell systems, the comparison of volumetric energy density with areal capacity (mAh/cm2), volumetric capacity (mAh /cc), and electrode swelling can only be guessed. Improving the volumetric energy density with only a Si anode is considered theoretically impossible due to the significant volume expansion of Si.
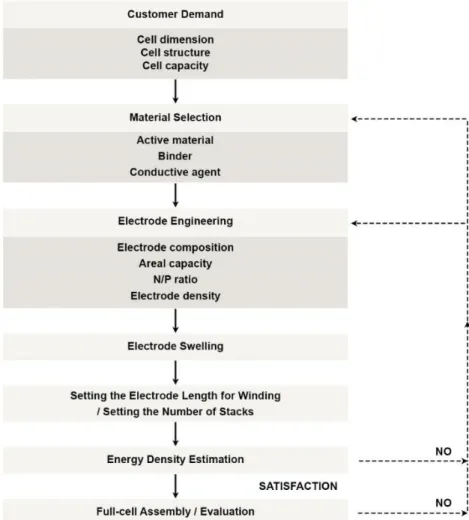
Research progress in co-utilization of graphite and Si
Due to the synergistic effect of the inactive matrix and the graphite in the composite, the composites showed stable cyclic behavior with a high specific power. Therefore, extensive studies on these variables are needed for the optimization of the graphite/Si composite.
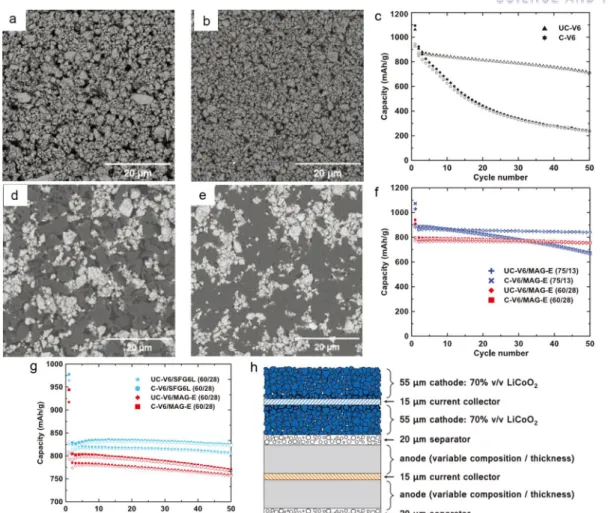
Critical factors for co-utilization of graphite and Si
A high Si content in the electrode usually has a lower full cell operating voltage. At the same Si content in the electrode, the swelling of the electrode strongly depends on the distribution of Si. Accordingly, material design is required to distribute Si homogeneously in the electrode to achieve low electrode swelling.
Regarding the graphite/Si composition, the distribution of Si in the electrode can be more homogeneous than that of Si anode mixed with graphite.
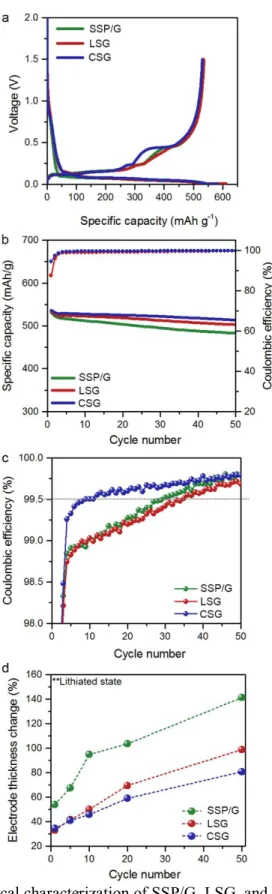
Conclusion and outlook
Our experimental verification demonstrates that the electrode swelling and electrochemical performance are strongly dependent on the Si distribution in the electrode. In summary, the homogeneously dispersed Si in the electrode leads to the mild electrode swelling compared to that of the localized Si in the electrode, which consequently affects the battery. To commercialize the graphite/Si anode, the stringent requirement for the electrochemical performance and the material price must be met.
The short-term goal for the graphite/Si anode is to achieve competitive electrochemical performances with the specific capacity of 500mAh/g compared to that of commercial graphite.
In this regard, we conclude that the electrode volume expansion is critical in determining the volumetric energy density of the full cell. Confronting issues of the practical implementation of Si anode in high-energy lithium-ion batteries. In this Perspective, we discuss key issues in the practical implementation of the Si anode in the high-energy full cell.
Accordingly, some recent papers have emphasized the use of the Si anode in a full cell and the consideration of the volumetric energy density 32, 33. In addition, we are dealing with performance fading of the Si anode in the full cell, which is less noticeable in the half cell. Depending on the volume of the swollen cell, the volumetric energy density of the silicon anode with graphite mixture decreases as the swelling of the electrode increases (Fig. 4. 4a).
In addition, the issue of electrode swelling is addressed in terms of the volumetric energy density. Meanwhile, we discussed the noticeable capacity fading of the Si anode in the full-cell.
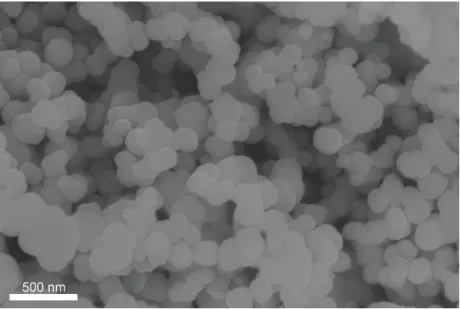
Micron-Sized Fe-Cu-Si Ternary Composite Anodes for High Energy Li-ion Batteries
Introduction
Experimental detail
In this Perspective, we discuss the key factors for the implementation of the newly developed Si anodes in the practical full cell. Finally, the low electrode density of the uncalendered electrode significantly lowers the volumetric energy density with a large fraction of voids in the electrode. In this regard, the measurement of the electrode swelling is just as important as the characterization of the.
For the practical application of Si anode in high-energy LIBs, it is imperative to investigate the electrochemical behavior and swelling of the Si anode electrode in the full-cell configuration by considering the design of the electrochemical cell.
Results and discussion
Conclusion
In summary, the new approach to solve the Si anode challenges is presented through the development of Si secondary structure containing void space and multiple nano-sized metal silicides (FeCuSi) by using scaled spray drying and simple handling of heat. Furthermore, regarding the full-cell test with LCO, FeCuSi had higher discharge capacity in each cycle during the 50th cycle with a high initial CE (89.4%) when compared to other comparison samples . The excellent performance of FeCuSi batteries is considered to be the result of distinctive features which were confirmed by the characterization of its material: 1) Crystallized Si nanoparticles from the amorphous one have several advantages in battery performance; 2) As a secondary structure with free space that enables smoothing of the volume change during cycling; 3) Abundant nano-sized metal silicides facilitate the improvement of electrical conductivity effectively through the reduction of interparticle resistance; 4) Those metal silicides can not only reinforce the secondary structure as an adhesive between Si particles, but also reduce the volume expansion of the composite through dilution of the lithium active phase. However, we realized that the cycling stability in the whole-cell system, under the conditions of high areal capacity and high electrode density, still needs to be further improved through adjusting the void space or wrapping the Si secondary structure with materials lithium-conductive and solid, such as coal tar.
We believed that this advanced secondary structure design with metal silicides, which can facilitate volume change and have a high electrical conductivity, will be a potential next-generation anode candidate for high energy density.
In this regard, before the practical implementation of the full-cell Si anode, the consideration of the electrochemical cell design is essential, just as for conventional LIBs. The average voltage, especially during discharge, is one of the most important parameters that contribute significantly to the energy density in the entire cell. Therefore, the extensive investigations are necessary for predicting practical electrode swelling in the cell, and the actual measurement of electrode swelling is essential.
The volumetric energy density varies as a function of cell thickness, and cell thickness strongly depends on electrode swelling and number of stacks.
One-to-one Comparison of Graphite-blended Negative Electrodes using Silicon
Introduction
Rechargeable Li-ion batteries (LIBs) are considered the most important energy sources for portable electronic devices, with a steady increase in energy density of 7–10% per year.1, 2 However, for future applications in vehicles electric vehicles (EV) and large-scale energy storage systems (ESS), achieving higher energy density is the most urgent priority in the LIB industry. 3-6 Considering the practical importance, Si-graphite blending has been investigated as a possible technique for next-generation negative electrode materials with improved energy density, 7-10 since the remarkable volume expansion of Si-dominant electrodes has hindered their application in practical batteries until now.11-13 In graphite mixed electrodes, graphite provides high electrical conductivity to the electrode components and allows electrode calendering to promote electrode density by acting as a lubricant.14 For more moreover, graphite increases the initial coulombic efficiency (CE) and cycling stability while mitigating the electrode volume expansion.7, 14, 15 For these advantages, the practical use of Si in anodes has been strongly studied through mixing with graphite. Here, we focused on the one-to-one comparison of the SGC hybrid anode material with state-of-the-art benchmark samples, including carbon-coated SiOx (denoted as C-SiOx) and a graphite/carbon containing Si composite ( denoted as Si–G/C), on graphite mixed electrodes. To our knowledge, few previous reports have suggested general electrochemical property tests of whole-cell configurations using industrial electrode requirements.27 Therefore, in this study, all electrochemical tests are performed with industrial electrode density (> 1, 6 g/cc ) and surface capacity (> 3.4 mAh/cm2), using a water-soluble styrene-butadiene rubber/carboxymethyl cellulose (SBR/CMC) binder compound (total 3.0 wt %) as in systems with both half and whole cells.
In addition, for a rational comparison, the specific reversible capacity of all samples is determined to be 420 mAh/g by mixing with graphite in different proportions, where the mixing ratios are 5 wt%, 19 wt% and 38 wt% for C-SiOx, Si-G /C and SGC, respectively.
Experimental detail
Then, in the cycling, the half cell was performed in a voltage range of 0.005-1V versus Li+/Li and the full cell was in the same voltage window as that of the formation stage. To measure the electrode volume expansion, the cells were disassembled in Ar-filled glove box and rinsed with dimethyl carbonate.
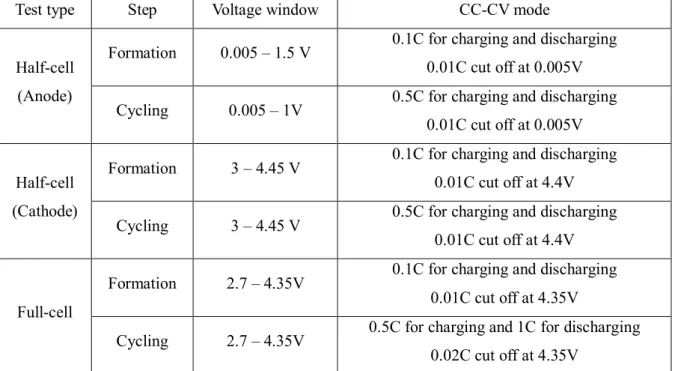
Results and discussion
3d–f plot the voltage profiles of the G/C-SiOx, G/Si-G/C and G/SGC electrodes corresponding to the above cyclic tests. Interestingly, for G/SGC, most of the capacity (3.1 mAh/cm2) is charged in the constant current (CC) section during the charging process, which is divided into CC mode and constant voltage (CV) mode. In order to elucidate the reasons for the different performances of the G/C-SiOx, G-Si-G/C and G/SGC battery, we investigated the macroscopic aspects of the electrode volume expansion (Fig.
For a more detailed identification of the morphology changes in the particles after cycling, we inspected the C-SiOx, Si-G/C and SGC particles in each individual graphite-blended electrode with SEM, EDS and TEM measurements, as shown in Figure 3.
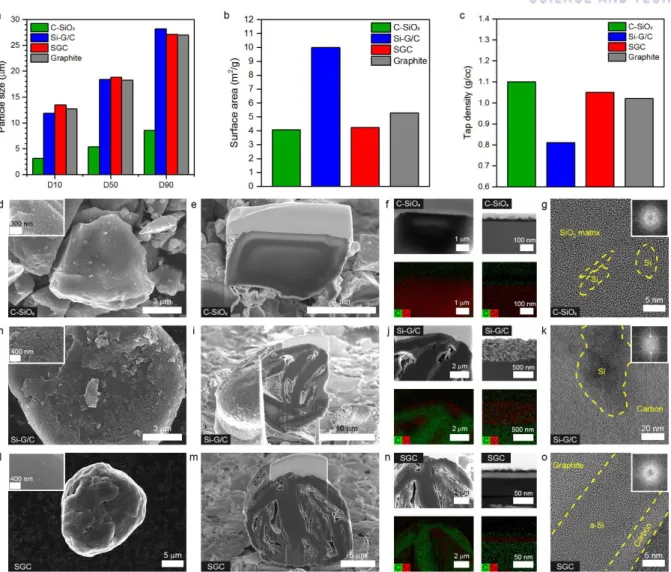
Conclusion
With the aim of the improvement in the volumetric energy density, the comprehensive overview of an electrochemical cell design for Si anodes is presented with its influence on. Typically, the electrode swelling strongly depends on the specific capacity of the anode due to the intrinsic property of anode materials33,52. The cyclable lithium is supplied infinitely from the lithium metal in the half-cell, while the supply is limited to the capacity of the cathode in the full-cell.
As the technological improvement of the Si anode progresses, the practical implementation of the Si anode in the high-energy full-cell is certainly the most important task for battery researchers.
Confronting Issues of the Practical Implementation of Si Anode in High-energy
Introduction
When it comes to the volumetric energy density, the electrode swelling during cycling is one of the most important factors to be mainly addressed. Ideally, the maximum energy density of the Si anode is achieved when its porosity becomes 0% in the fully lithiated state. When it comes to the practical application of the Si anode, the electrode swelling is one of the most critical challenges due to the volumetric energy density, cycling performance and safety.
For the sake of low electrode swelling, the volume expansion of Si should be investigated in terms of particles and electrode levels.
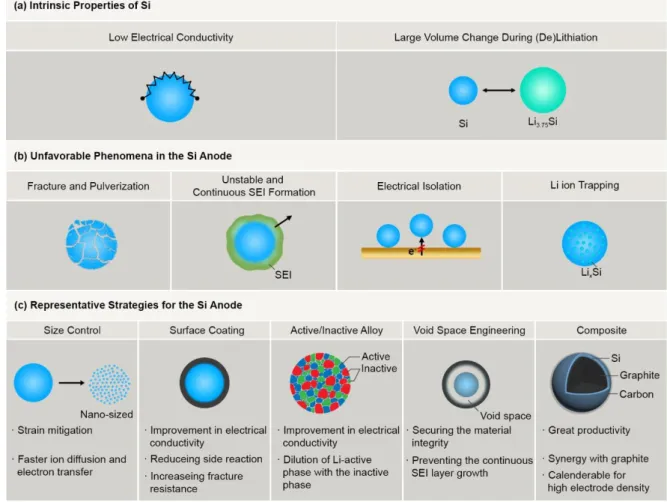
Results and discussion
Conclusion and outlook
For a precise assessment of the Si anode, the influence of the electrochemical cell design on the electrochemical properties is discussed in detail. At the electrode level, Si and void distribution is important for electrode swelling. As we have emphasized in this perspective, the electrochemical properties of the Si anode are highly dependent on the design of the electrochemical cell, and capacity degradation is accelerated in a full cell.
We believe that the extensive consideration on the design of electrochemical cells, which specifically focuses on high volumetric energy density, will lay the foundation for the realization of high energy LIBs using the Si anode.
Dash, R.; Pannala, S., Theoretical limits of energy density in silicon-carbon composite anode lithium-ion batteries. Ko, M.; Chae, S.; Cho, J., Challenges in tailoring the volume change of Si anodes for lithium-ion batteries. Zhao, H.; Yuan, W.; Liu, G., Hierarchical design of high-performance alloy nanomaterial electrodes for lithium-ion batteries.
W.; Yan, K.; Zhuo, D.; Lin, D.; Liu, N.; Cui, Y., LixSi nanoparticles protected by artificial solid electrolyte interphase: An efficient and stable paralithiation reagent for lithium-ion batteries.