Sn 3d XPS spectra of (a) pristine Sn4P3 anodes and after precycling in (b) baseline, (c) FEC-added and (d) FEC+TMSP-added electrolyte. P 2p XPS spectra of (a) pristine Sn4P3 anodes and after precycling in (b) baseline, (c) FEC-added and (d) FEC+TMSP-added electrolyte. C 1s XPS spectra of (a) pristine Sn4P3 anodes and after precycling in (b) baseline, (c) FEC-added and (d) FEC+TMSP-added electrolyte.
Introduction
The Na-ion batteries
Although lithium-ion batteries are already marketed in portable devices, the price of lithium resources is rising rapidly due to its low presence in the Earth's crust. Therefore, sodium-ion batteries (NIBs) have attracted much attention for large-scale electrical energy storage due to their low cost compared to lithium-ion batteries. Although the application of Na-ion batteries still has a long way to go, it has recently reported a number of new materials and tracked the performance of Li-ion batteries.
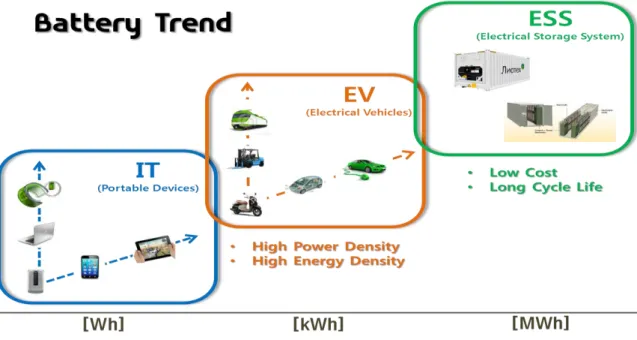
1-2.Theoretical background
In particular, these additives change the surface chemistry of SEI layer in metal anode and reduce surface resistance due to the formation of thin SEI layer.27 In the case of cathode, boron-based addition shows improvement of cycle performance due to the suppression of the oxidation reaction in high voltage.28-29 In addition, additives such as flame retardants that can reduce gas generation have a key issue for battery safety. When SEI layer is formed by oxidation of additives for cathode, the additives must be high HOMO energy. In the case of anode, the additives must be lower LUMO energy because additive must reduce reaction to make SEI layer on the anodes.
2-1. Introduction
Cyclic carbonate-based electrolytes improving the electrochemical performance of Na4Fe3(PO4)2(P2O7) cathode for sodium-ion batteries. However, when the electrode materials reach their performance, interface reactions between electrolytes in batteries must be considered. Therefore, reducing the dissolution of electrolyte and making a stable surface layer formed on the cathode determines the coulombic efficiency and long-term cycle performance.
In this work, we demonstrate key aspects of electrolyte formulation to afford high electrochemical performances of the Na4Fe3(PO4)2(P2O7) cathode. Electrochemical Performance of Cathodes with Different Electrolytes Furthermore, we investigated the products of the electrochemical reaction at the cathode by ex-situ X-ray photoelectron spectroscopy (XPS) and the reactivity of the electrolytes towards the Na metal electrode by 13C NMR techniques.
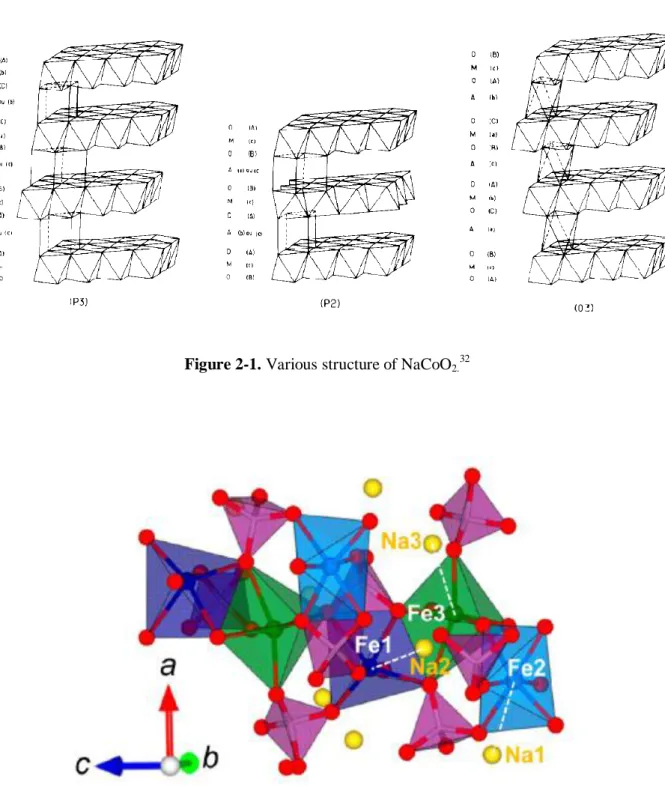
2-2. Experiment Section
13C NMR spectra of the electrolytes contacting the Na metal electrode were recorded on an Agilenet spectrometer (VNMRS 600). After 10 days, each of the electrolytes was carefully obtained in the argon-filled glove box and injected into the NMR tube with THF-ds solvent. The surface morphology of the electrodes was observed with a field emission scanning electron microscope (FE-SEM; JEOL JSM-6700F).
After the pre-cycling process, the cells were carefully opened in a glove box to recover their electrodes. The electrodes were then rinsed in dimethyl carbonate to remove the residual NaClO4-based electrolyte, and the resulting material was dried. Samples were prepared in a glove box and sealed with an aluminum foil pouch under a vacuum before use.
The samples were then quickly transferred to the chamber of the XPS instrument to minimize potential contamination.
2-3. Results and discussions
2-6(a) displays the ionic conductivities of EC/DEC- and EC/PC-based electrolytes as a function of NaClO4 salt at room temperature. The comparison of the oxidation stability of EC/DEC- and EC/PC-based electrolytes is shown in Fig. This result indicates that EC/PC/1M NaClO4 electrolyte is more stable at high voltage condition compared to EC/DEC/1M NaClO4.
Cathode with EC/DEC/1M NaClO4 exhibits significant overcharge with increasing voltage plateau length at around 3.2 and 4.1 V vs. The significant overcharge behavior of the cathode in EC/DEC/1M NaClO4 led to drastic reduction of the initial coulombic efficiency of 418. %. A picture of spacers and Na metal electrodes obtained from Na4Fe3(PO4)2(P2O7)/Na half-cells after precycling in EC/DEC/1M NaClO4 and EC/PC/1M NaClO4 is shown in the inset of Fig.
In contrast, the separator and the Na metal electrode cycled in EC/DEC/1M NaClO4 showed a strong color change (inset in Fig. In addition, the cathode with EC/DEC/1M NaClO4 had two voltage plateaus (regions A and B) that were not observed for the EC/PC-based electrolyte (Fig. These results also show that the EC/PC-based electrolyte is less reactive than EC/DEC towards the Na metal electrode (region A) and is relatively stable at high voltages (region B).
This is because very thick SEI layer formed by decomposition of EC/DEC/1M NaClO4 blocks the P signal from the cathode.
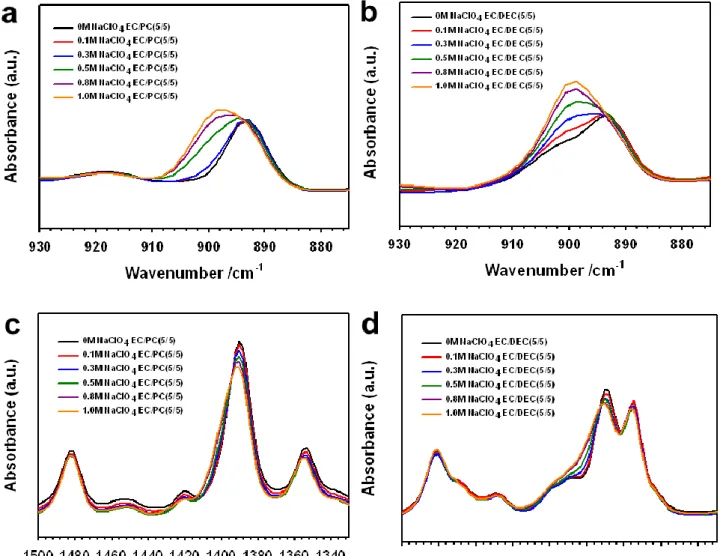
2-4. Conclusion
3-1. Introduction
Interface architectures based on a combination of binary additives for high-performance Sn4P3 anodes in sodium-ion batteries. Although Sn4P3 exhibits high theoretical capacity and low redox potential, it experiences severe volume expansion during cycles. This volume expansion due to Na insertion and extraction causes the anode particles to burst and leads to continuous electrolyte decomposition on the exposed active surface of the anode.46 The electrolyte decomposition immediately causes thick solid electrolyte interphase (SEI) filming and results in increasing interphase resistance.
To improve the cycle performance, the architecture of stable and robust protective layer on the metal anode is extremely important in NIB. The significant process of SEI formation depletes the limited Na+ source in a cell, makes thick SEI layers provoking the loss of electrical conduction pathways in the electrode, and results in poor cycling performance. Therefore, it is already well known in LIBs that functional additives make the SEI layer stable and greatly improve cycle performance.
To understand the interfacial characteristics and electrochemical reactions of Sn4P3 anode with and without additives, surface morphology observations, spectral and XRD studies of Sn4P3 anode should be needed.
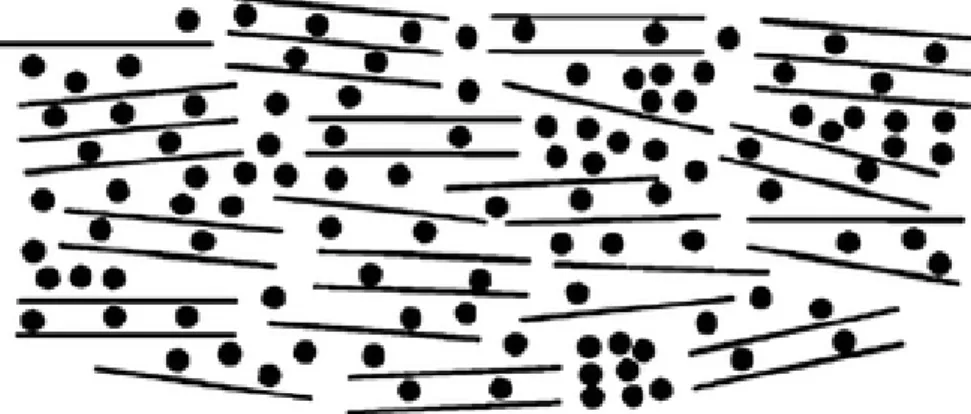
3-2. Experimental Section
3-3.Result and Discussion
To further confirm the origin of the B peak observed in the baseline electrolyte, ex situ XRD patterns of the Sn4P3 anodes before and after the precycle were obtained, as shown in Figure 3-8. This means that the Na15Sn4 phase is easily produced in the base electrolyte, compared to the FEC and FEC+TMSP added electrolyte. Interestingly, the presence of FEC in the electrolyte led to the formation of Na9Sn4 instead of Na15Sn4, as shown in the XRD pattern of Figure 3.8(a).
Ex-situ Indeed, the aggregation of anode particles between 1 and 2 µm occurred after the precycle in the baseline electrolyte, as shown in Figure 3-9(a),(b). On the contrary, no significant agglomeration of anode particles was observed for the anode precycled in the FEC- and FEC+TMSP-added electrolyte (Figure 3-9(c),(d)).
In particular, the anode precycled in the FEC+TMSP-containing electrolyte maintained the original morphology of anode particles very well. This result suggests that good electrical connection in the anode with the binary additive FEC+TMSP is maintained during cycling. This HF removal can reduce NaF formation in the SEI of the Sn4P3 anode.
Evidence for the presence of Si-F and Si-O groups in the SEI layer on the anode pre-in the FEC+TMSP-added electrolyte is given in the Si 2p spectra of Figure 3-12(d).
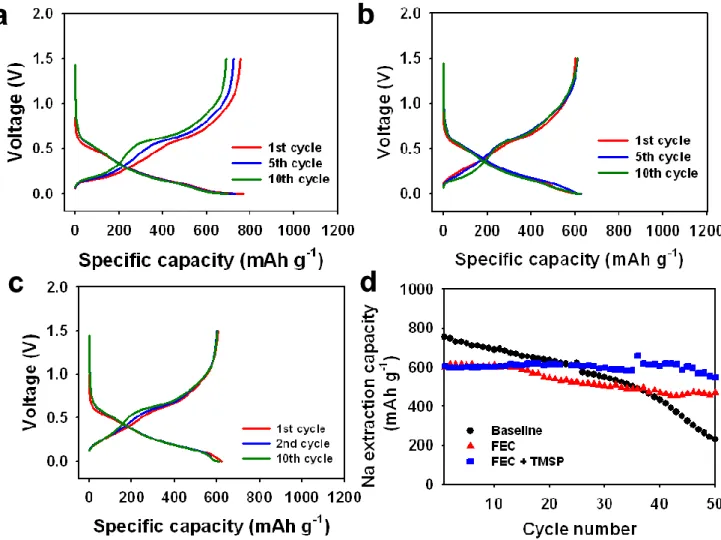
Increase of SOC*
The C 1s XPS spectra of Figure 3-14(b) clearly show that the peaks assigned to ether (C-O-C) moiety and sodium carbonate (Na2CO3) are more intense for the Sn4P3 anodes cycled in the baseline electrolyte becomes, compared to the FEC and FEC+TMSP containing electrolyte. This result is in good agreement with the EDS results that strong C, O and Cl signals formed by the electrolyte decomposition are detected on the anode precirculated in the baseline electrolyte. It is clear that the FEC and TMSP additives effectively prohibit the formation of ether and Na2CO3 through the EC decomposition, as shown in Figure 3- 14(c),(d).
In addition, comparison of the C 1s XPS spectra of Figure 3-14(b)-(d) shows that the peak corresponding to C-C of carbon black particles is significantly reduced in the case of basic electrolyte. This decrease in the intensity of the C-C peak is attributed to a very thick surface film formed by the decomposition of the base electrolyte that blocks the C-C signal from the carbon black particles. This result is consistent with the SEM observation showing the formation of the SEI layer on carbon black (area A of Figure 3-9 (b)).
A remarkable feature is that the interfacial resistance, including the SEI resistance and charge transfer resistance components, is higher for the Sn4P3 anodes with full sodium sodium in the FEC and FEC+TMSP added electrolyte compared to the base electrolyte. This finding indicated that the NaF produced by FEC decomposition acts as a high-resistivity layer to form the Na15Sn4 phase, which exhibits a strong volume increase. The half-cycle of the Sn4P3/Na half-cells with FEC+TMSP after complete sodition was slightly smaller than the FEC-added electrolyte anode, probably because the TMSP additive modified the NaF-based SEI layer.
Electrochemical impedance spectra of Sn4P3 anodes (a) before and (b) after complete baseline sodium oxidation, with FEC added and with FEC + TMSP added electrolyte.
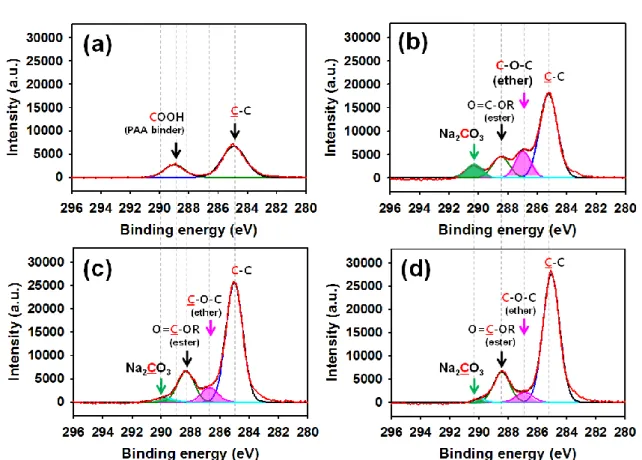
3-4 Conclusion
S.; Cho, J., A highly cross-linked polymeric binder for high-performance silicon negative electrodes in lithium-ion batteries. Kovalenko, I.; Zdyrko, B.; Magasinski, A.; Hertzberg, B.; Milicev, Z.; Burtovyy, R.; Luzinov, I.; Yushin, G., A key component of brown algae for use in high-capacity lithium-ion batteries. B.; Carretero-Gonzalez, J.; Rojo, T., Na-ion batteries, recent advances and challenges to become low-cost energy storage systems.
H.; Ceder, G.; Kang, K., Electrode Materials for Rechargeable Sodium-Ion Batteries: Potential Alternatives to Current Lithium-Ion Batteries. C.; Fasching, R.; Aurbach, D., Effect of Fluoroethylene Carbonate (FEC) on the Performance and Surface Chemistry of Si-Nanowire Lithium-Ion Battery Anodes. Komaba, S.; Yabuuchi, N.; Nakayama, T.; Ogata, A.; Ishikawa, T.; Nakai, I., Study on the reversible electrode reaction of Na1-xNi0.5Mn0.5O2 for a sodium-ion rechargeable battery.
S.; Jeon, S.; Kang, K., Novel Iron-Based Mixed Polyanion Cathodes for Lithium and Sodium Rechargeable Batteries: Combined First-Principles Calculations and Experimental Study. S.; Kang, K., Understanding the Electrochemical Mechanism of a Novel Iron-Based Na4Fe3(PO4)(2)(P2O7) Mixed Phosphate in a Na Rechargeable Battery. T.; Fraisse, B.; Stievano, L.; Monconduit, L., Better cycling performance of bulk Sb in Na-Ion batteries compared to Li-Ion systems: An unexpected electrochemical mechanism.