Especially with the development of electric vehicles and smart grid system, interest in energy storage system with high energy density and security is increasing. Until now, lithium-ion batteries (LIBs) have been responsible for this energy storage system. However, LIBs have limitations in terms of high prices and safety issues to be used as a large-scale energy storage system.
Na0.69Fe2(CN)6 and Fe2(CN)6 were tested as cathode material for the magnesium-based system. Na0.69Fe2(CN)6 showed small and reversible structural change and stable cycling performance over 30 cycles. Comparing Na0.69Fe2(CN)6 and Fe2(CN)6, it appears that the existence of Na+ aids the reversible intercalation/de-intercalation of magnesium.
In this report, magnesium intercalation phenomena in Na0.69Fe2(CN)6 and natural graphite were reported. Because these are the first reports for magnesium-based system, they are expected to introduce a new way of magnesium system research.
Introduction
- Lithium ion batteries
- Magnesium based batteries
- Cathode materials for Mg 2+ ion storage
- Anode materials for Mg 2+ ion storage
Because there were no electrolytes that can be operated in the high voltage range, the development of cathode materials was also limited in the low voltage range near 2 V (relative to Mg/Mg2+), the need for high voltage cathode materials becomes greater.10 Second, magnesium metal cannot be used together with conventional polar aprotic solvents.11 When metals or electrodes meet electrolytes, SEI (Solid Electrolyte Interface) layers form on the surface. Other kinds of cheveral materials such as Mo6T8 (T = S, Se) were reported as cathode materials.9d, 9e Cheveral phase cathodes delivered around 100 mA h g-1 with stable cycle performance.
MoO3 and MnO2 were introduced as cathode materials.14 They showed higher specific capacity than chevron phase materials but showed poor cycle performance stability. These cathode materials were operated in the low voltage region (< 2 V vs. Mg/Mg2+) due to the absence of high voltage electrolytes. Here, I introduce one of the Prussian blue analogs, Na0.69Fe2(CN)6, as a magnesium-retaining cathode material.
Analogues of Prussian blue have been reported as cathode materials for lithium and sodium ion batteries.16 Most of them can be easily synthesized by a simple precipitation method, which means easier use in large-scale production. In addition, due to their cubic structure and large channel size, they have great potential as cathode materials.
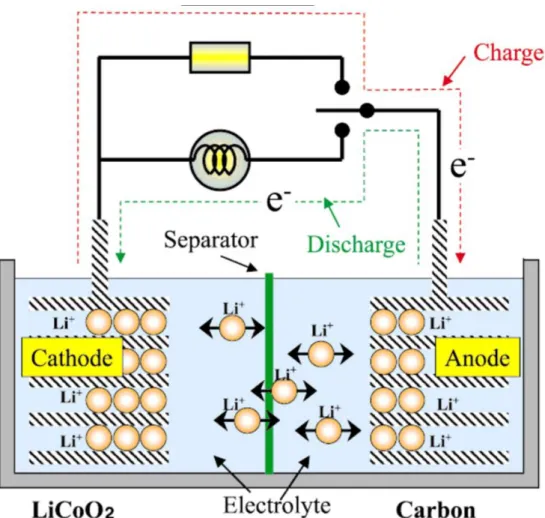
Experimental
- Synthesis
- Material characterization
- Electrode preparation
- Cell configuration
- Electrochemical test
Active material and conductive carbon were first mixed with mortar and mixed with binder solution. After that, the mixture was mixed with mixing machine (Thinky ARE-250, Interelectronics Co.) with extra addition of NMP for appropriate viscosity. Counter electrode was prepared with activated carbon (MSP-20), conductive carbon (Super P) and binder (PTFE: polytetrafluoroethylene).
The sheet was dried at 120 °C and dried again immediately before cell assembly at the same temperature. The open circuit voltage of activated carbon is measured with Ag/Ag+ reference electrode several times.
Results and discussion
- Preparation of materials
- Electrochemical properties
- Structural and oxidation state analysis
- Reversible Mg 2+ intercalation
This means that not only inserted Mg2+ ions but also existing Na+ ions in bare NFCN acted as charge carriers. To confirm that existing Na+ ions can act as charge carriers, electrochemical properties of NFCN were tested starting with the charging process. This means that both existing Na+ ions in NFCN and inserted Mg2+ ions from electrolyte acted as charge carriers.
Moreover, regardless of starting discharge or charge, NFCN delivered approximately 70 mAh h g-1 after the second cycle. Structural change of FCN and NFCN with Mg2+ ions was investigated by ex situ .
On the other hand, in the case of NFCN, the XRD peak shifted reversibly during the discharge and charge process. After Mg2+ ions were first intercalated into NFCN (discharge), the XRD peak at 17–18° shifted to a lower angle, meaning the lattice of NFCN became larger. Because not only the inserted Mg2+ ions but also existing Na+ ions were removed, the XRD peak shifted to a higher angle than the original NFCN state.
According to the intercalation and decomposition of charge carriers, the lattice parameter of NFCN changed reversibly. When the amount of cations in NFCN increases, the structure of NFCN starts to distort and changes to the rhombohedral structure. So, the variance of the lattice parameters is greater in the unloading process than the loading process due to the phase transition to the rhombohedral structure.
Then the peak shifted to high energy in the next charge, which means Fe was oxidized after the charge process. This results from the decomposition of Na+ in the charge process consistent with ex situ XRD analysis. Reversible interference and de-interference of Mg2+ ions were investigated by ex situ TEM analysis.
After Mg2+ ions were intercalated into NFCN, Mg was clearly detected in NFCN particles by EDS mapping. This phenomenon comes from the faster diffusion kinetics of Na+ ions than that of Mg2+ ions.
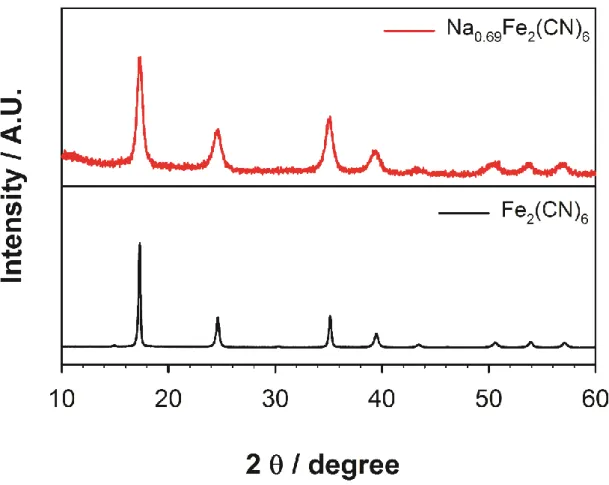
Mg 2+ co-intercalation into Natural graphite
Experimental
- Material characterization
- Electrode preparation
- Cell configuration
- Electrochemical test
As shown in Figure 21, natural graphite showed reversible cycling with a constant capacity of 180 mAh g-1 at a current density of 2 mA g-1. As shown in the SEM images in Figure 22, the surface of the magnesium anode after several cycles is different from the intact magnesium anode. This indicates that magnesium has actually participated in the electrochemical reaction and stripping and deposition of magnesium occurs at the magnesium anode.
In order to investigate detailed structural changes, natural graphite was examined by TEM analysis after magnesium intercalation. The co-intercalation of magnesium into natural graphite is supported by ex situ Fourier transform infrared (FT-IR) analysis. It indicates that the solvated magnesium ions were co-intercalated with the solvents into the native graphite during the discharge process.
Reversible structural change was observed by ex situ XRD during magnesium intercalation and de-intercalation. This indicates that 0.09 mol of magnesium was reversibly decomposed with the existing sodium to Na0.69Fe2(CN)6. Due to the bivalence of magnesium ions, the coulomb attraction between the Mg-(CN) bond is greater than the Na-(CN) bond.
This affects the kinetics of magnesium deintercalation, resulting in greater deintercalation of sodium than magnesium. A reversible structural change was observed by ex situ XRD during intercalation and deintercalation. 002) peak of natural graphite disappeared with intercalation and recovered with subsequent deintercalation. There were points where the (002) layers of natural graphite collapsed, and magnesium ions were mostly detected in this region.
To confirm the participation of magnesium in the reaction, SEM analysis of the magnesium metal surface was performed. He showed that there were peaks of electrolytes in the electrode which means co-interference of solvents in natural graphite. The intercalation of magnesium in Prussian blue compounds with sodium and the co-intercalation of magnesium in natural graphite with ethereal solvents may suggest a new method for the development of magnesium-based battery systems. a) Dunn, B.; Kamath, H.; Tarascon, J.-M., Electric energy storage for the grid: A battery of choices.
Results and discussion
- Electrochemical properties
- Magnesium anode analysis
- Structural analysis
- Co-intercalation of magnesium with solvent
Conclusion
In this study, magnesium ion intercalation materials were introduced for electrode materials for magnesium-based battery systems. One of the Prussian blue analogs, Na0.69Fe2(CN)6, is introduced as a cathode material that can store magnesium ions. The possibility of sodium deintercalation can be confirmed with an electrochemical test of Na0.69Fe2(CN)6 starting with the charge.
Comparing Fe2(CN)6 and Na0.69Fe2(CN)6, it appears that the existence of sodium allows magnesium ions to be more easily intercalated in Prussian blue analogues. To confirm the co-intercalation of solvents in natural graphite, ex situ FT-IR was performed with a magnesium intercalated electrode. Na0.69Fe2(CN)6 and natural graphite were introduced as materials for magnesium ion intercalation electrodes that can be used as cathode and anode, respectively.
Perrin, M.; Poggi, P., Assessment of world lithium resources and consequences of their geographical distribution on the expected development of the electric vehicle industry. Han, D.-W.; Lee, K.-H.; Lee, S.-S.; Doo, S.-G., High-capacity nanostructured manganese dioxide cathode for rechargeable magnesium ion batteries.