Analysis of thermal stress in advanced materials based on non-Fourier theories of heat conduction has become a popular topic in recent decades. 7 lists some perspectives on future developments in non-Fourier heat conduction and thermal stress analysis.
Introduction
Heat Conduction
Fourier Heat Conduction
It is worth mentioning that Fourier heat conduction Eq. 1.1) and the first and second laws of thermodynamics in Eq. 1.6) needs modifications for the heat transport analysis of thermoelectrics. An isolated boundary condition is a special form of Eq. c) The convection heat transfer with ambient and radiation heat exchange with a radiator occurs at the interface:. kTrTðx;tÞjx¼S¼ h Tð ðx;tÞ.
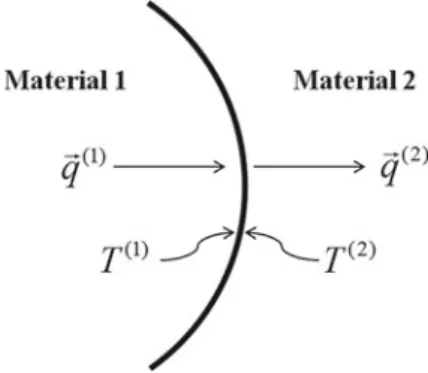
Non-Fourier Heat Conduction
Several types of extension of the Taylor series can be considered to develop the TPL thermal conductivity equations. The other types of TPL heat conductivity can also be developed by Taylor series extension of Eq.
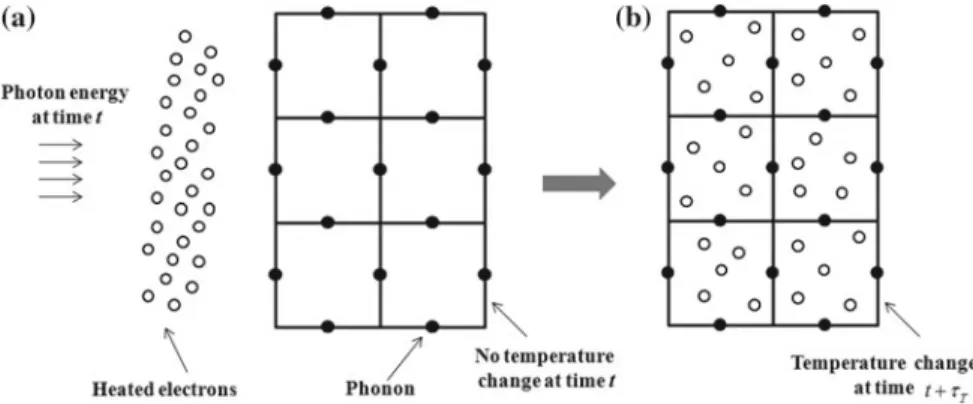
Moisture Diffusion
Fickian Moisture Diffusion
The negative sign in Eq. 1.38) implies that moisture is distributed in the direction of decreasing moisture concentration. The Fickian transient moisture diffusion differential equation is derived by substituting Eq. r:ðkmrmðx;tÞÞ þqrmðx;tÞ ¼@mðx;tÞ.
Non-Fickian Moisture Diffusion
In the absence of VðxÞ, Eq. 1.47) corresponds to the C-V model for non-Fourier heat conduction. Akbarzadeh A, Chen Z (2012) Heat conduction in one-dimensional functionally graded media based on the dual-phase-layer theory.
Introduction
Laplace Transform and Laplace Inversion
Fast Laplace Inverse Transform
Durbin used the fast Fourier transform (FFT) to speed up the computation time of Laplace inversion. To minimize the numerical discretization and truncation errors, it is recommended to consider the following constraints on the arbitrary parameters:
Reimann Sum Approximation
In addition, a is an arbitrary real number greater than all real parts of the singularity present in the function f xð ;sÞ and L and Nare are two arbitrary parameters that affect the accuracy of the solutions.
Laplace Inversion by Jacobi Polynomial
To minimize numerical discretization and truncation errors, it is recommended to consider the following restrictions for arbitrary parameters: where is a positive real number db[ 1. Moreover, Pðna;bÞ represents the Jacobi polynomial of degree, defined as:.
Non-Fourier Heat Conduction in a Semi-in fi nite Strip
Figure 2.2 compares temperature distribution at non-dimensional tidb¼1 for classical Fourier, C-V, diffusive-like DPL, wave-like DPL and NL C. The effect of non-dimensional correlation length L on temperature distribution is illustrated in Fig. 2.4, at non-dimensional time b¼1 using the NL C-V heat conduction model.
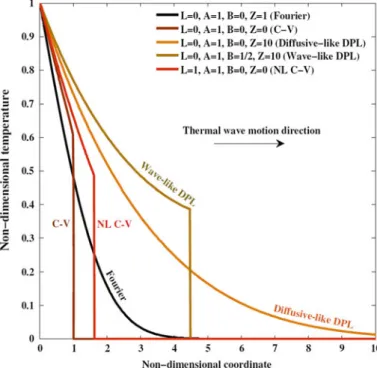
Nonlocal Phase-Lag Heat Conduction in a Finite Strip
Molecular Dynamics to Determine Correlating
Similar to the temperature profile observed in a nano-argon film [12], temperature in the nanoplate evolves in the form of thermal wave. Due to the importance of FGMs, we present here the temperature evolution and thermal wave propagation in an FGM nanoplate.
![Fig. 2.10 A nano-slab considered for MD simulation of thermal wave propagation [7]](https://thumb-ap.123doks.com/thumbv2/123dok/10290055.0/49.659.86.564.90.246/fig-nano-slab-considered-simulation-thermal-wave-propagation.webp)
Three-Phase-Lag Heat Conduction in 1D Strips, Cylinders,
Effect of Bonding Imperfection on Thermal Wave
The thermal excitation causes the heat wave to propagate towards the inner surface of the cylinder. While the temperature is discontinuous at the interface of the two-layer cylinder, the radial heat flux is continuous at the interface, which is compatible with the thermal boundary conditions considered in Eq.
![Fig. 2.14 Effect of thermal compliance on the distribution of a temperature and b heat- fl ux at dimensionless time f ¼ 0 : 126 ð m ¼ 1 ; e 0 ¼ 0 : 35 ; d 0 ¼ 0 : 25 ; Hyperbolic DPL Þ [8]](https://thumb-ap.123doks.com/thumbv2/123dok/10290055.0/58.659.137.527.89.616/fig-effect-thermal-compliance-distribution-temperature-dimensionless-hyperbolic.webp)
Effect of Material Heterogeneity on Thermal Wave
The sandwich plate is perfectly bonded ðvT ¼0Þ and is made of inner and outer Cu layers, while the middle layer of the plate is Cu, Ag or Au. If the time increases, the heat wave will be transmitted and reflected from all interfaces and reflected back from the inner and outer surfaces of the plate to finally reach the steady state temperature.
Thermal Response of a Lightweight Sandwich Circular
A decrease in the relative density in the middle layer increases the transition temperature in the middle and outer layers of the cylinder, while it is. The heat flow in the cylinder decreases with the relative density of the middle layer [8].
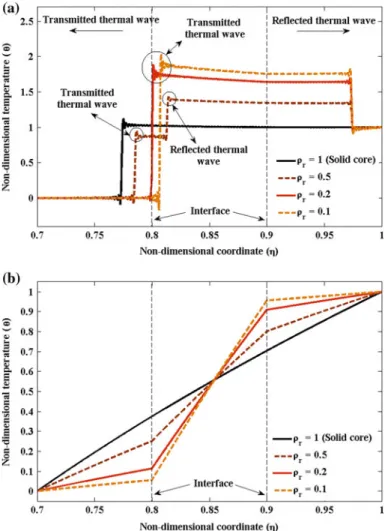
Dual-Phase-Lag Heat Conduction in Multi-dimensional Media
DPL Heat Conduction in Multi-dimensional Spherical
Akbarzadeh A, Chen Z (2013) Heat conduction in one-dimensional functionally graded media based on double phase shift theory. Akbarzadeh A, Chen Z (2012) Transient heat conduction in a functionally graded cylindrical plate based on double phase shift theory.
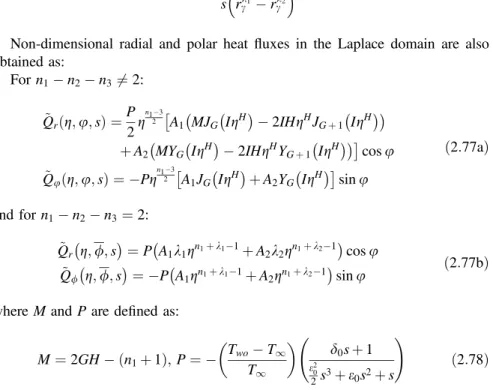
Smart Materials
Piezoelectric Materials
Di¼eijkejkþeijEjþci#þvim; ði;j;k;l¼1;2;3Þ ð3:1Þ in which rijandDiare voltage and electric displacement;eij,Ek,#and mare deformation, electric field, temperature change and moisture concentration change with respect to the reference state; Cijkl, ekij and ij are elastic, piezoelectric, dielectric and coefficients; bij, nij, ci and vi are thermal stress, hygroscopic stress, pyroelectric and hygroelectric coefficients. Furthermore, #¼ TT0 and m=MM0, where T and M are the absolute temperature and moisture concentration, while T0 and M0 represent the stress-free temperature and moisture concentration.
Magnetoelectroelastic Materials
However, Biot [23] introduced the effects of the elastic term in the energy equation to obtain more accurate results for thermoelastic analysis. The following Fourier theory of heat conduction which relates the heat flux to the temperature gradient is the most widely used theory in the literature: qi¼ kTij#;j ð3:19Þ Moreover, the diffusion of moisture in a solid body is essentially i same as temperature.
Advanced Smart Materials
The conventional heat conduction and moisture diffusion theories based on the classical Fourier and Fickian laws lead to an infinite rate of thermal and moisture wave propagation due to the parabolic heat and mass transport equations. Accordingly, various non-Fourier and non-Fickian heat and mass transfer theories have been developed to overcome these drawbacks.
Thermal Stress Analysis in Homogenous Smart Materials
Solution for the a Thermomagnetoelastic
Equation (3.27) can be rewritten in the following form: r2u;rrþrð2Nþ1Þu;rþð2NdaÞuþr2W;rr. rð2Nþ1ÞkW;rþr2YH;rþrð2Nþ1ÞYH¼0 ð3:30bÞ. Equation (3.40) and its derivative are replaced by the first equation of (3.38) and give us the following decoupled differential equation for you: . 3.41) can be obtained exactly as follows. 3.41);A,B andCare the integration constants determined by the boundary conditions; and Kiði¼1;2;3Þare obtained as:.
Solution for Thermo-Magnetoelectroelastic
F are new integration constants and. kcf2ðK1ðcð1þmÞ fð1þbÞÞ aC1ðXfYcÞÞ;. ð ÞðcfÞ þðK2þK1ÞðmcbfÞ aC2ðXfYcÞ K1ðcð1þmÞ fð1þbÞÞ. Considering the expressions for u and W, one can obtain the following expression for using Eq. U¼HþGlnð Þ þq Bl0ðlnð Þq Þ2þCl1qmþDl2qmþðl3lnð Þ þq l4ÞqþK3l5q3 ð3:63Þ whereGandH are integration constants and.
Benchmark Results
The dimensionless temperatures on the inner and outer surfaces of the FGM hollow cylinder are assigned Ha and Hb. Furthermore, the dimensionless magnetic induction in Figure 3.4c increases as the internal temperature increases.
![Table 3.2 Material properties of BaTiO 3 / CoFe 2 O 4 [33, 34]](https://thumb-ap.123doks.com/thumbv2/123dok/10290055.0/97.659.246.580.465.886/table-material-properties-batio-cofe-o.webp)
Thermal Stress Analysis of Heterogeneous Smart Materials
Solution Procedures
To simplify the analysis, we introduce the following non-dimensional parameters:. ð3:77Þ as well as a new electric potential and temperature change as follows:. Make a variable change ofq¼e1, Eq. 3.80) is converted to the new differential equations with constant coefficients in the following form:
Benchmark Results
The voltages, electric potential, electric displacement, magnetic field perturbation, and temperature distribution are given for various non-dimensional magnetic fields, inertial effects, aspect ratios, and non-homogeneity parameters, as well as various non-dimensional values of indoor and outdoor temperatures. Figure 3.6a–d shows the effects of non-dimensional magnetic field X on the distribution of stresses, electric displacement and electric potential along the radial direction of the hollow FGPM cylinder with N¼ 1, angular velocityxn¼1 and aspect ratioi¼4 under Case 1 boundary conditions in the above equations without any thermal disturbance.
Effect of Hygrothermal Excitation on One-Dimensional Smart
- Solution Procedure
- MEE Hollow Cylinder
- MEE Solid Cylinder
- Benchmark Results
The effect of hygrothermal boundary conditions on the multiphysical responses of a MEE hollow cylinder is depicted in Fig.3.8. Furthermore, increasing the temperature and moisture concentration on the outer surface reduces the magnetic potential through the thickness of the cylinder as shown in Fig.3.8e.
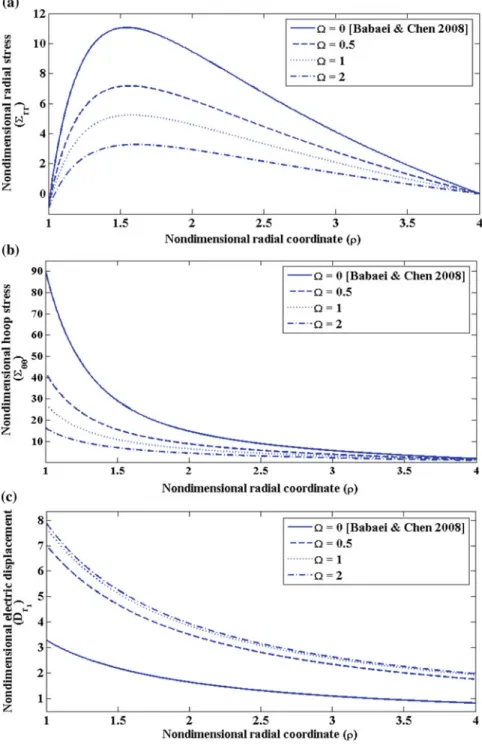
Remarks
Yas MH, Shakeri M, Khanjani M (2011) Layer-by-layer finite element analysis of a functionally graded hollow thick cylinder with a piezoelectric ring. Babaei M, Chen Z (2008) Analytical solution for the electromechanical behavior of a rotating functionally graded piezoelectric hollow shaft.
Functionally Graded Materials
By properly choosing the degree of variation of the composite materials, it is possible to essentially control (increase or decrease) the temperature of the material when subjected to excessive thermal stress [4]. The design of an FGM with external ceramic properties and internal thermal conductive properties, as well as a properly chosen degree of gradation could greatly reduce the temperature within the walls of the spacecraft and reduce the risk of failure [2,4].
Hyperbolic Coupled Thermopiezoelectricity
- Introduction
- Homogeneous Rod Problem
- Solution Procedure
- Results and Discussion
Figure 4.3 shows the temperature change at non-dimensional moments¼0:1848 along the length of the rod. In addition, the positivity of the electric potential changes along its length before the heat source leaves the rod.
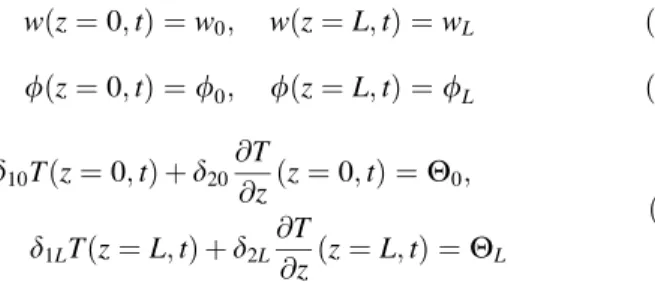
Hyperbolic Coupled Thermopiezoelectricity in Cylindrical
- Introduction
- Hollow Cylinder Problem
- Solution Procedure
- Results and Discussion
This section emphasizes the effects of the inhomogeneity index and the thermal relaxation time on the results. In this section, we analyze the effects of both the inhomogeneity index and thermal relaxation time on the cylinder response to thermal shock.
![Fig. 4.10 The element, local coordinate and shape functions used in the current section [17]](https://thumb-ap.123doks.com/thumbv2/123dok/10290055.0/150.659.243.578.84.237/fig-element-local-coordinate-shape-functions-current-section.webp)
Coupled Thermopiezoelectricity in One-Dimensional
Introduction
The Laplace transform and the state-space approach allowed the solution of the governing partial differential equations [31]. In the following section, the governing equations for the problem are given in general forms, which include the generalized coupled (Lord-Shulman and Green-Lindsay), classical coupled and classical uncoupled thermoelasticity theories.
The Functionally Graded Rod Problem
In particular, a more general formulation is sought to understand the coupled and uncoupled behavior of the functionally graded piezoelectric medium at hand [3]. The governing equations are written below in a general form for the analysis according to the coupled and uncoupled thermoelastic theories of a piezoelectric rod rij¼cijkleklbijðhþvhÞ eijkEk ð4:47aÞ Di¼eijkeijkþpiðhþvhÞ þ 2ijEj ð4:47bÞ. The variables and constants in these equations were previously defined in Section 4.2.2 and are omitted here.
Solution Procedures
Applying the boundary condition;/;@h@xx¼0. l¼ð0;0;0Þon the system results in the following system of equations which can be solved for the integration constants:. Also, the normalized stress and electrical displacement can be found in the Laplace domain as shown below:
Results and Discussion
Therefore, there are distinguishable smooth sections of the rod in the temperature and stress distribution. The time history of the difference between the coupled and uncoupled theory temperature solutions is shown in Figure 4.20.
![Table 4.2 Material properties of the left end of the rod [3, 31]](https://thumb-ap.123doks.com/thumbv2/123dok/10290055.0/166.659.247.578.89.327/table-material-properties-left-end-rod.webp)
Introduction of Dual Phase Lag Models
Moreover, the heat flux retardation may not be identical to the temperature gradient retardation [40]. Giving the phase delays in this structure also allows us to investigate the generalized thermoelasticity based on the Lord-Shulman (L-S) theory.
Results of Dual Phase Lag Model Analysis
In the following two figures, the effect of the temperature gradient phase delay sh¼t1 on the temperature is studied for the C-T theory with t26¼0 and t2¼0. However, the locations of the wavefronts remain the same for varying values of inhomogeneity.
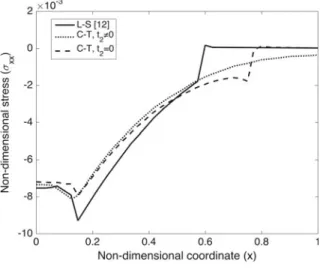
Remarks
Babaei M, Chen Z (2009) The transient coupled thermopiezoelectric response of a functionally graded piezoelectric hollow cylinder to dynamic loadings. Akbarzadeh A, Babaei M, Chen Z (2011) Coupled thermopiezoelectric behavior of a one-dimensional functionally graded piezoelectric medium based on C-T theory.
Introduction
Extended Displacement Discontinuity Method and Fundamental
Fundamental Solutions for Unit Point Loading
Then the corresponding displacements and stresses are obtained, for example the stresses of an arbitrary point in the upper domain are obtained as: and the forms of the stresses in the Cartesian coordinates are: For example, the stresses and heat flux of an arbitrary point in the upper domain are given as:
![Fig. 5.1 A penny-shaped crack with radius of a lying in the interface plane [39]](https://thumb-ap.123doks.com/thumbv2/123dok/10290055.0/184.659.132.521.89.304/fig-penny-shaped-crack-radius-lying-interface-plane.webp)
Boundary Integral-Differential Equations
Similar to Section 5.2.1.3, since the problem is symmetric with respect to the x and y axes, under a unit point displacement discontinuity in the x direction the stresses of any point in the upper domain are obtained in Cartesian coordinates. In the vicinity of point P, for a small SR, displacements and temperature discontinuities are related only to the x-coordinates in the normal plane through the point Pas, as well as to the position of the point Pa along the crack outline [33,36].
![Fig. 5.3 The local intrinsic coordinate system at the interface crack front [39]](https://thumb-ap.123doks.com/thumbv2/123dok/10290055.0/198.659.256.577.88.426/fig-local-intrinsic-coordinate-interface-crack.webp)
Stress Intensity Factor and Energy Release Rate
Interface Crack Problems in Thermopiezoelectric Materials
Basic Equations
Fundamental Solutions for Unit-Point Extended
Boundary Integral-Differential Equations for an
Hyper-Singular Integral-Differential Equations
Solution Method of the Integral-Differential
Extended Stress Intensity Factors
Fundamental Solutions for Magnetoelectrothermoelastic
Fundamental Solutions for Interface Crack Problems
Fundamental Solutions for Unit-Point EDDs
Application of General Solution in the Problem
Summary
Introduction
Hyperbolic Heat Conduction in a Cracked Half-Plane
Basic Equations
Temperature Field
Temperature Gradients
Numerical Results
Thermoelastic Analysis of a Partially Insulated Crack
De fi nition of the Problem
Thermal Stresses
Asymptotic Stress Field Near Crack Tip
Numerical Results and Discussions
Thermal Stresses in a Circumferentially Cracked Hollow
Problem Formulation
Thermal Axial Stress in an Un-cracked
Thermal Stress in the Axial Direction
Stress Intensity Factors
Results and Discussion
Transient Thermal Stress Analysis of a Cracked Half-Plane
Formulation of the Problem and Basic Equations
Solution of the Temperature Field
Solution of Thermal Stress Field
Numerical Results and Discussion
Summary
Heat Conduction Theories
Application in Advanced Manufacturing Technologies