The output voltage and current density of the sponge-structured TENG are measured in the forward connection and the reverse connection. Calculated electrostatic potentials of Au nanoparticle-embedded mosoporous TENG with increasing concentration of Au NPs simulated by COMSOL multi-physics software.
INTRODUCTION
Both the voltage and current output of the TENGs are proportional to the triboelectric charge density on the surface [36, 37], so that the output power has a quadratic dependence on the charge density. Moreover, different types of nanostructures ensure highly flexible, stable and high output performance of the nanogenerators mainly based on the porous nanostructured platforms.
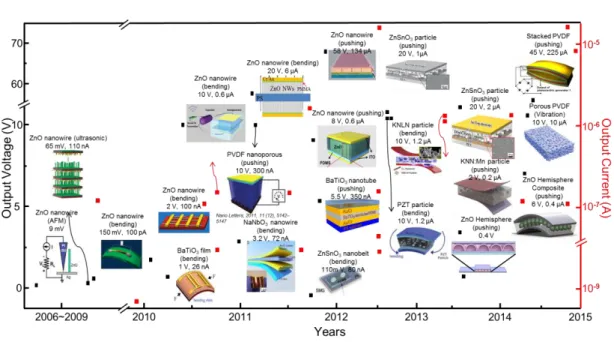
THEORETICAL BACKGROUND AND MOTIVATION
Piezoelectricity
- Introduction to piezoelectricity
- Piezoelectric energy harvesting
The wide range of piezoelectric materials allows their use in a variety of diverse applications. The output power of a piezoelectric nanogenerator largely depends on the impedance of the circuit or load connected to the piezoelectric nanogenerator.
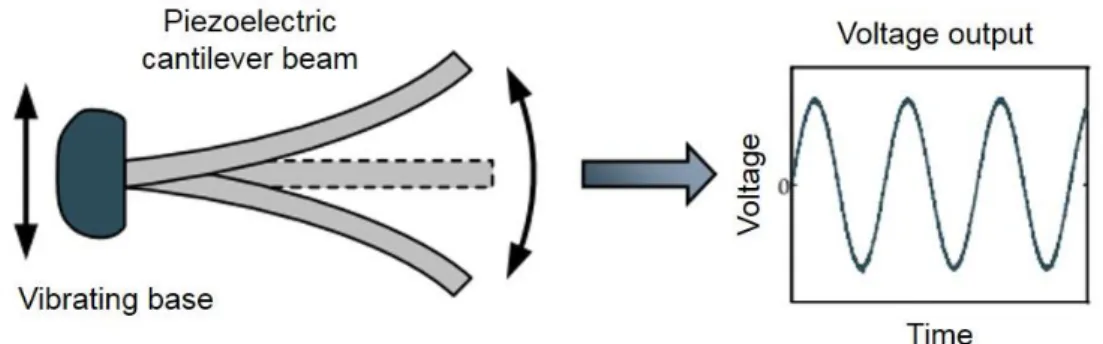
Triboelectricity
- Mechanism for contact charging and charge transfer
- Triboelectric energy harvesting
The amount of charge transferred is equal to the product of the contact potential difference and the capacitance between the two bodies. If we define the electric potential of the lower electrode (UBE) to be zero, the electric potential of the upper electrode (UTE) can be calculated with.
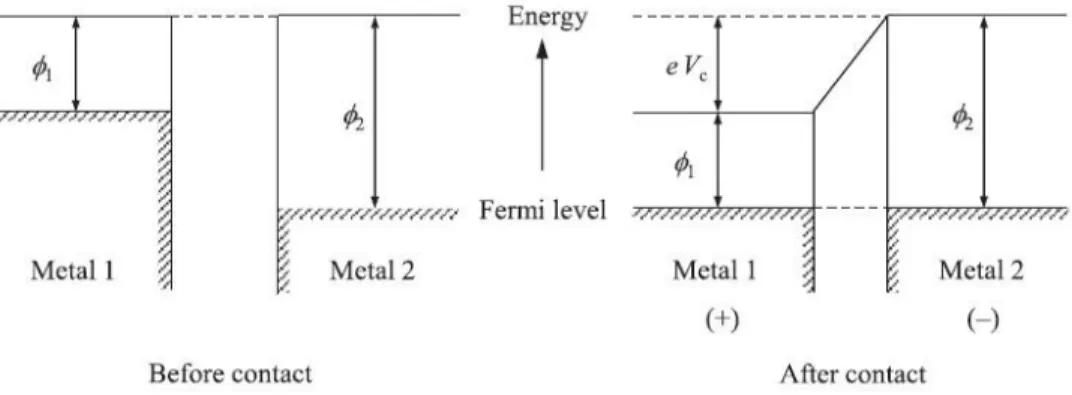
OBJECTIVE AND IDEAS
Flexibility for stable output performance
Difference in work function between two materials for electron transfer
Thus, the potential difference with the Fermi level of the bottom electrode will increase the output capacity of the TENG. Calculated charge density and (b) output voltage under control of the work function between the metal and the dielectric material in separation equilibrium.
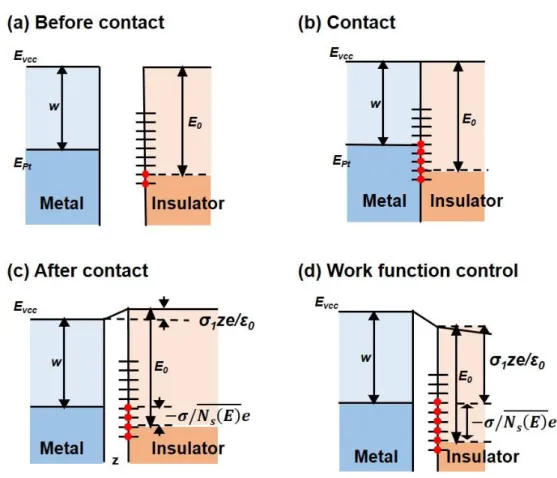
Dielectric constant of insulating layer for high surface charge density
Thus, I calculate the potential difference and short-circuit current by increasing the dielectric constant (ε) and the capacitance change (dC/dt) per unit time of the polymer in the TENG. Calculated potentials and (b) short-circuit current with increasing dielectric constant and change in polymer capacitance in the TENG.
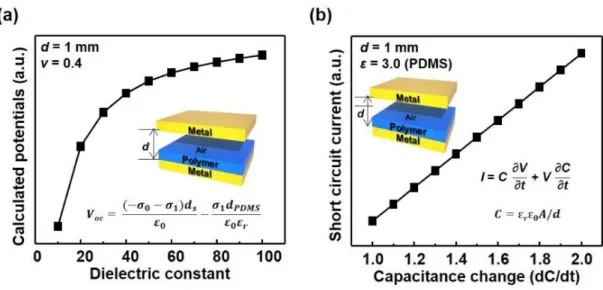
EXPERIMENTAL PROCEDURE
- Embossed hollow hemispheres thin films
- Pressure Sensor
- Piezoelectric nanogenerator
- Piezoelectric hemispheres with stretchable composite films
- Highly ordered sponge structured films
- Mesoporous Pores Impregnated with Au Nanoparticles
- Measurement of electrical characteristics
- Microstructural analysis
As a top electrode, ITO layer was then placed above the heads of the embossed ZnO films. A nanogenerator was also made by stacking two pieces of the hemispheres. a) Schematic diagrams of the pressure sensor manufacturing process. The SiO2/Si substrate was treated with UV/Ozone (AHTECH LTS, South Korea) to make the surface of the substrate hydrophilic.
A nanogenerator was also made by stacking two pieces of the structures. a) Schematic diagrams of the manufacturing process for the piezoelectric hemispheres embedded stretchable composites. This slow drying process is very important to get the nanoparticles to the bottom of the pore (Figure 15). To fabricate nanogenerator, the 10 μm-thick PDMS film was fabricated on the Al electrode attached by the double-sided polyimide tape, as shown in the schematic fabrication process of the TENGs (Figure 16a).
Schematic fabrication process of mesoporous films with embedded Au NPs. a) Schematic diagrams of the fabrication process of the top and bottom electrodes of a mosoporous TENG embedded with Au nanoparticles without an air gap. Cross-sectional optical and SEM images of Au nanoparticle-embedded mosoporous TENG without air gap (b). The crystalline properties of the films were characterized using a high-resolution X-ray diffractometer (Bruker, Germany).
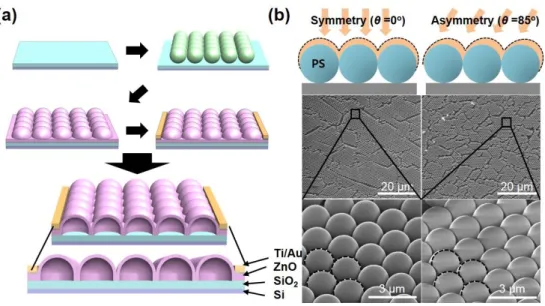
RESULTS AND DISCUSSION
Highly responsive pressure sensor based on embossed hollow hemisphrere
- Crystal characteristics
- Output performance
- Numerical analysis
- Polarity test
Embossed hollow hemisphere-based piezoelectric nanogenerator
- Output performance
- Stacking method
By pushing the nanogenerator, the imprinted film with symmetric hemispheres repeatedly generates an open circuit voltage of ~0.2 V, which is the same voltage in the imprinted film with asymmetric hemispheres. The simulation results along the vertical direction are clearly seen in Figure 22b. a) Open circuit voltage and (b) piezoelectric potential distributions of nanogenerators with symmetric (top) and asymmetric (bottom) hollow hemispheres. It was performed by spin-coating the polystyrene on the hemispheres, followed by deposition of ZnO film and post-annealing.
The layer-by-layer integrated nanogenerator shows. the improved output voltage of ~ 0.4 V as the sum of the output voltage of ~ 0.2 V from the two individual hemispheres, as shown in Figure 23. -circuit voltage of embossed thin film generator with single-layer and two-layer hollow hemispheres. a) Output voltage and (b) current density, generated by the ZnO hemisphere embedded composites as a function of the diameter of the hemispheres from 0.5 to 10 m and.
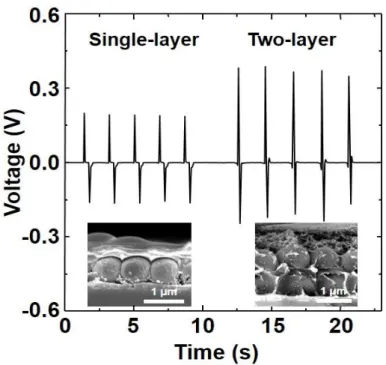
Piezoelectric hemispheres with stretchable composite nanogenerator
- Output performance
- Numerical analysis
- Mechanical test
- Stacking method
- Anisotropic output characteristics
The piezoelectric response phase and deformation amplitude were obtained on top of the PZT hemispheres. The simulation in Figure 24e supports that as the hemisphere diameter increases, the displacement of the hemisphere along the vertical direction increases, resulting in a larger piezoelectric potential. When applying a vertical compressive force per unit area of the composite, the number of hemispheres in the composite is reduced with a large hemisphere diameter, and the load is concentrated in the hemisphere due to the load confinement effect.
By linearly fitting a loading curve up to 40% engineering strain, the elastic modulus of the PDMS film and the composite film are calculated to be 1.13 MPa and 0.91 MPa, respectively. Although the rupture stress of the ZnO halves layer in the composite film is much smaller than that of the PDMS films, the adhesion strength between the ZnO halves and the PDMS matrix in the composite film is quite high. As the number of hemisphere layers increases, the output voltage and output current density increase to 6.0 V and 0.2 μA/cm2, respectively, at three. layer-stacked nanogenerator, as shown in Figures 28a and 28b.
The simulation result in Figure 28c, which shows the increase of the piezo potential as the number of stacking layers increases, supports the increase of the electrical outputs with the number of layers. To investigate the decrease in the electrical outputs, the same electrical measurement was performed with the direction of the bend, convex and concave. Therefore, the position of the hemispheres plays an important role in improving the output power.
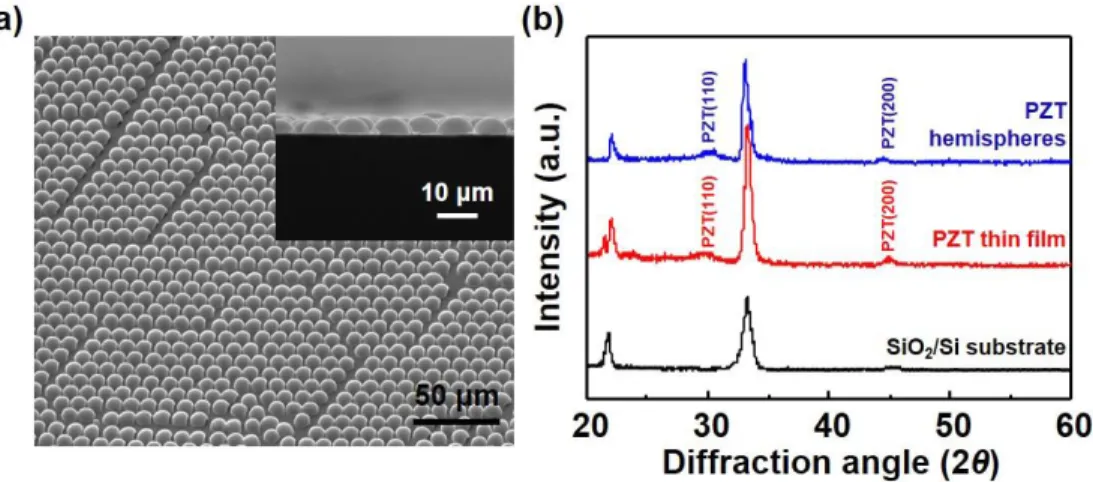
Hydrophobic sponge structure-based triboelectric nanogenerator
- Microstructural analysis
- Output performance
- Stability test
- Force-response capacitance change
- Mechanical test
- Numerical analysis
In general, the charge generation of the sponge-structured TENG and film-structured TENG under cyclic compression force can be understood from the coupling of the triboelectric effect and electrostatic induction. The stability and durability test of the sponge-structured TENG, the output voltage of the 0.5 µm diameter-based sponge-structured TENG under cyclic compressive force. In detail, the dramatic increase in the electrical output performance of the sponge-structured TENG can be attributed to the increase in the surface area-to-volume ratio of the sponge-structured PDMS films.
Therefore, the larger ratio increases the contact area, which larger area further increases the surface charges during the contact of the films, which increases the total output power in the sponge-structured TENG. The output voltage and current density of the film-structured TENG and sponge-structured TENG and 10 µm), with the applied forces from 30 to 90 N. I further measured the output voltage and current density from the 1, 3, 5, and 10 µm pore diameter-based sponge-structured TENGs under the same compressive force.
To investigate the correlation between the electrical output performances of the sponge-structured TENG with contact forces, a systematic measurement under different pressure forces was also performed. As shown in Figure 35, under a contact force of 30 N, the maximum output voltage generated by the sponge-structured TENG (0.5 µm) is 15 V. 29] Thus, in the original state, the capacitance of the flat film is larger than that of the sponge-structured film.
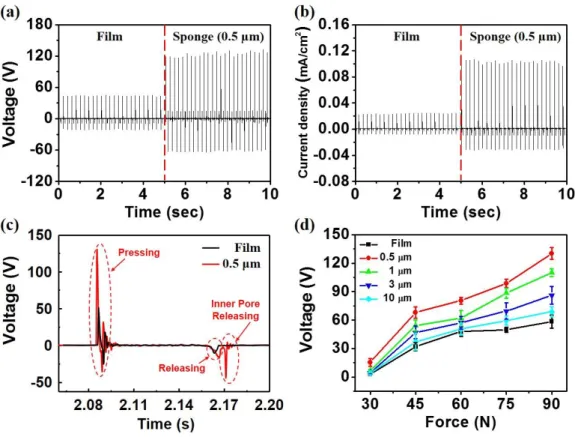
Au nanoparticles-embedded mesoporous triboelectric nanogenerator
- Porosity-response compressibility
- Output performance without airgap
- Mechanical test
- Output optimization
- Porosity-response capacitance change
- Numerical analysis
- Output performance with airgap
The output voltage and (b) current, generated by the Au nanoparticles-embedded mesoporous TENG as a function of Au NPs concentrations from 5.8 to c) Charge generation mechanism of the Au nanoparticles-embedded mesoporous TENG under external power at short circuit condition. Charge generation mechanism and measurement systems of the Au nanoparticles embedded mosoporous TENG under external power at open circuit condition. The output voltage (a) and output current density (b) of the Au nanoparticles-embedded mosoporous TENG under external power during 360 s.
The pores in the mesoporous film play a very important role in the electrical output performance of mesoporous TENGs embedded in Au nanoparticles. This simple, gap-free design enables a sealing process in the device, at the edges of the Au nanoparticle-embedded mosoporous TENG, and at the surface of the top and bottom electrodes. It is clearly seen that the output voltage drop rate and current density are significantly reduced with sealing.
It is obvious that the output voltage and current density reach a record value of 220 V and 2 µA/cm2 from the Au nanoparticle-embedded mosoporous TENG, where the output performance of the TENGs also increases with the Au content. The output power of the Au nanoparticle-embedded mosoporous TENG was also measured with external loads from 10 Ω to 1 GΩ, as shown in Figure 51a. The rectified output voltage and current density and (b) the rectified output power of the Au nanoparticle-embedded mosoporous TENG without a gap with the resistor.
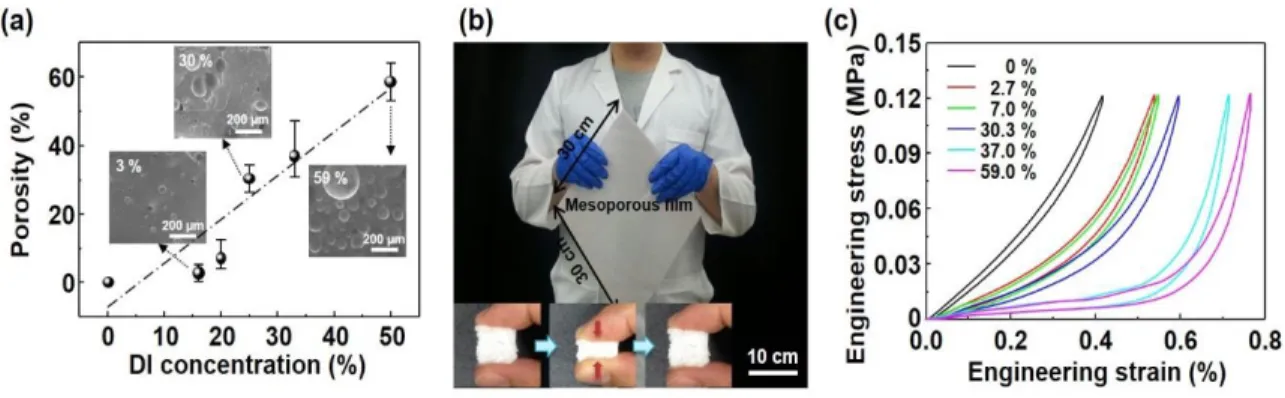
Applications in self-powered devices
- Motion sensor for health monitoring
- Hydrophobicity based humidity sensor
- Shape mapping sensor for monitoring the local touching
- Foot-step driven energy harvester
- Battery charging system
The electrical output performance of the film-structured TENG and the sponge-structured TENG based on different pore sizes was measured and plotted under different relative humidity conditions, as shown in Figure 54a. Performance characterization of the film-structured TENG and sponge-structured TENG under different humid atmospheres. a) The change in output voltage with change in relative humidity for flat and sponge structure films with a pore size of 10 µm. An apparatus to investigate the effect of humidity on the output performance of the TENGs.
To demonstrate the effect of humidity on the performance of the sponge-structured TENG, I used directly series-connected LEDs from the sponge-structured TENG under different humid atmospheres. The above results confirm that the surface modification of PDMS from the flat film to sponge-structured film can dramatically improve the performance of the TENG over a wide range of humid atmospheres, and therefore it is less sensitive to humidity and produces stable electrical output performance. Contact angles of the DI water on the (a) flat film and (b) 1 μm sponge-structured film. c) Water meniscus and the net force on sponge structure overhang (Wt > Wb).
Higher power produced higher saturation voltage of the capacitor, which is a result of equilibrium establishing the charge rate and the capacitor leakage rate for the Au nanoparticles embedded mesoporous TENG. The electrical output performance of the sponge-structured based TENG increases with decreasing diameter of pore size, which is attributed to the rapidly increased contact area. The improvement is believed to be attributed to the increase in the density of charges created by the contact between Au NPs and PDMS within the pores, thereby affecting the surface potential energy of mesoporous films.
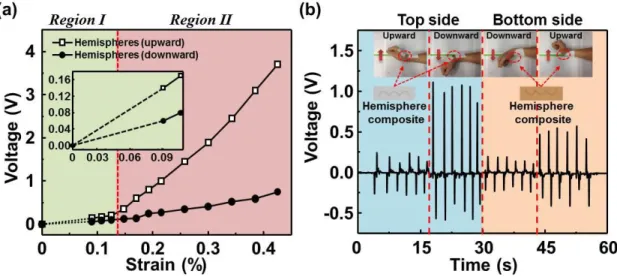
CONSLUCION