Sangmin Park School of Materials Science and Engineering Ulsan Graduate School National Institute of Science and Technology. Among nanocrystals, magnetic nanoparticles are hot topics due to their wide uses for applications such as catalysis, nano-medicine, absorption of electromagnetic waves, bio-sensors and data storage. In the first part of the paper, I briefly presented the general description of magnetic nanoparticles including magnetism, properties, synthetic method.
Iron cobalt (FeCo) nanoparticles are one of the important magnetic nanoparticles due to their large values of permeability and saturation magnetization. During the synthesis, hydrogen gas was injected directly throughout the reaction time, leading to the reduction of the oxygen portion of the nanoparticles. I also synthesized size and shape controlled FeCo nanoparticles by controlling the amount and ratio of oleic acid and oleylamine.
There are many attempts to fabricate nanoparticles/graphene composites by EDC coupling, in-situ fabrication and so on. In the second method, the surface of FeCo nanoparticles organic ligands are exchanged into inorganic ligands to give the negative surface charge, while PEI-coated graphene oxide has positive charge. Metal oleate precursors have a high boiling point and decomposition of the precursors produces monodisperse nanoparticles.
Schematically illustrated procedure for the synthesis of FeCo nanoparticles with hydrogen gas a) flowing and b) directly injected into the reaction mixture, and their X-ray diffraction patterns. Beam patterns of as-synthesized magnetic nanoparticles: a) hydrogen gas directly injected into the reaction mixture with the low flow rate b) using phenyl ether as solvent.
Theoretical background of magnetic nanoparticles
- Introduction
- Magnetism
- Diamagnetism
- Paramagnetism
- Ferromagnetism
- Antiferromagnetism
- Ferrimagnetism
- Properties of magnetic nanoparticles
- Size effect
- Shape and structure effect
- Surface effect
- Composition effect
- The synthesis of magnetic nanoparticles
- Overview
- Thermal decomposition
- Co-precipitation
- Hydrothermal synthesis
- Microemulsion
One is the anisotropy energy which can be explained by KV (V is the domain volume) and domain wall motion. If the size of the magnetic nanoparticles is smaller, the Ms of the magnetic particles also decreases due to the spin effect. In the case of a cobalt nanorod with dimensions of 15 nm with an aspect ratio of 10, the coercivity of the nanorod is 4500 Oe at room temperature.25.
Because of the surface-to-volume ratio, external spins are an important factor that can regulate magnetic properties. As the particle size decreases, the magnetization of the particles decreases due to surface effects compared to the bulk. There are many possible reasons such as magnetic insulating layer on the surface, tilted spin, spinning glass like behavior of surface spins.
When the surface of nanoparticles is modified, such as absorbing different molecules, the value of Keff. So the net magnetic force of Fe3O4 comes from the magnetic moments of Fe2+. The net magnetization of the nanoparticles can be tuned by replacing Fe2+ with Mn2+, Co2+ or Ni2+.
Controlling the size, morphology, composition of the particles is important for the use of magnetic nanoparticles for applications such as biomedicine, data storage, because the various properties of the nanoparticles depend on the parameters. Many magnetic nanoparticles such as Fe37,38, Co39,40 and Ni41 monodisperse nanoparticles can be synthesized through the method.42,43 Another method is referred to as the "heating" method. Although the method is easy and can be carried out under ambient conditions, it has some disadvantages compared to the thermal decomposition method.
The geometry and magnetic properties of the nanoparticles can be tuned by varying experimental factors such as temperature, salt, injection currents, presence of oxygen, ionic strength and pH, etc.46. In this method, the morphologies such as the size and shape of the nanoparticles can be adjusted by parameter such as temperature, reaction time, concentration of reactant, solvent, precursors, etc. The size of the micelles can be controlled by controlling the ratio between their compositions.49 If the desired reactants are added to water-in-oil microemulsions, a precipitate forms in the micelles.
So the size of the MnFe2O4 nanoparticles can be controlled by controlling the ratio of water to toluene.50. Because they have monodispersivity and are easy to control morphology such as size and shape of the magnetic nanoparticles.
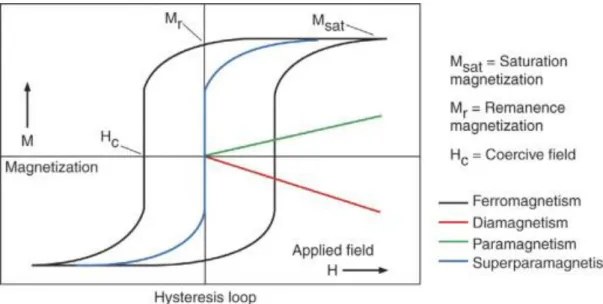
The synthesis of size- and shape-controlled FeCo nanoparticles
- Introduction
- Experimental method
- Result and discussion
- Reduction of FeCo with hydrogen gas
- Synthesis of various size and shape of FeCo nanoparticles
- Conclusion
The synthesized FeCo nanoparticles were precipitated by adding 15 mL of ethanol and centrifugation (6000 rpm, 5 minutes) and dissolved in hexane. There are several reports regarding the role of hydrogen in preventing FeCo nanoparticles from oxidation. Schematically illustrated synthesis procedure of FeCo nanoparticles with hydrogen gas a) flowing and b) injected directly into the reaction mixture and their X-ray diffraction patterns.
For the further research, I injected the hydrogen gas directly into the reaction mixture during the synthesis procedure. We checked that as-synthesized FeCo nanoparticles by the method of hydrogen gas flow do not contain little oxygen. On the contrary, FeCo nanoparticles by the method of direct hydrogen gas injection contained low level oxygen fractions, which means that hydrogen gas reduces the oxygen fractions of FeCo nanoparticles.
But in the case of phenyl ether there was no sign of FeCo (figure 2.2b), but CoFe2O4 did inject hydrogen gas. Oxygen of ether group prevents the formation of FeCo.. jet patterns of as-synthesized magnetic nanoparticles: a) hydrogen gas injected directly into reaction mixture with the low flow rate b) using phenyl ether as a solvent. The carboxyl group (-COOH) on OA and amine group (-NH2) on oleylamine have different strength and selective binding energy with the surfaces of the nanoparticles. 62-65 OA is known to bind selectively and strongly to the crystalline facets like a (100) plane.
Thus, iron and cobalt sources can be used for nanoparticle growth, leading to an increase in nanoparticle size. For deeper investigations of surfactants, I reduced the total amount of surfactants to 1/3, resulting in 5 nm FeCo nanoparticles (Figure 2.5). During synthesis, OA molecules are attached to the lowest-energy {100} facets and inhibit nanoparticle growth in the [100] direction by forming a dense surfactant layer.66-68 Since this crystal plane is blocked, metal ions are absorbed on {111} or {110} aircraft.
Low-oxygen FeCo nanoparticles are formed as hydrogen gas which is injected directly into the reaction mixture. Various size and shape of FeCo nanoparticles were also synthesized varying the amount and ratio of oleic acid and oleylamine. A diverse range of applications such as bio-application, data storage, electromagnetic wave shielding, biomedical products will be more developed with the various FeCo nanoparticles.
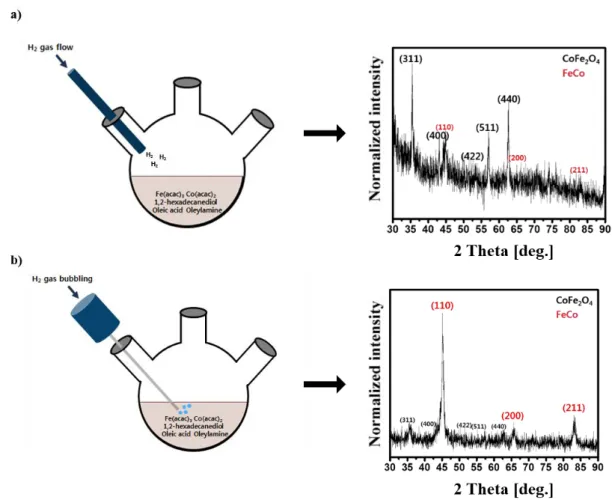
Attachment magnetic nanoparticles to edge-oxidized graphene
- Introduction
- Experimental method
- Result and discussion
- Attachment CoFe 2 O 4 nanoparticles to EOG by EDC coupling
- Attachment FeCo nanoparticles to EOG by electrostatic interaction
- Conclusion
And the temperature of the mixture was increased to 100oC and this temperature was maintained for 10 minutes. 40 mg of the as-synthesized CoFe2O4 nanoparticles were dissolved in 4 mL of toluene, followed by addition of a solution containing 40 mg of DMSA and 4 mL of DMSO. And the temperature of the reaction mixture was raised to 60°C and this temperature was maintained for 12 hours.
Ligand exchange of FeCo nanoparticles with Cl ligands and formation of FeCo/EOG composites. 40 mg of FeCo nanoparticles (4 mg/ml) was added to a vial containing 200 mg of NH4Cl dissolved in 10 ml of DI water. The immiscible two-phase mixture was stirred vigorously until the phase transfer of FeCo nanoparticles from the upper hexane phase to the lower DI water phase was completed. This washing process was repeated three times to completely remove the unreacted Cl-ligands, and the Cl-capped FeCo nanoparticles were redispersed in 10 mL of DI water.
To attach Cl-capped FeCo nanoparticles to edge-oxidized graphene, the nanoparticles and EOG were mixed at a ratio of 8:1. One of the advantages of EDC coupling is that the reaction can be performed in water, making the reaction is possible without the aids of organic solvent. EDC and NHS are added to the mixture of PEI-EOG and DMSA-coated CoFe2O4 nanoparticles for chemical bonding of the composites.
Unlike CoFe2O4 nanoparticles, it is difficult to fit DMSA on the surface of FeCo nanoparticles. To form the FeCo/EOG composite, I just mixed Cl-capped FeCo and PEI-EOG in water. The FeCo nanoparticles were attached to the edge of the EOG, suggesting the formation of composites.
Gu, H.; Zheng, R.; Zhang, X.; Xu, B., Facile One-Pot Synthesis of Bifunctional Nanoparticle Heterodimers: Conjugate of Quantum Dot and Magnetic Nanoparticles. Yang, H.; Zhang, J.; Tian, Q.; Hu, H.; Fang, Y.; Wu, H.; Yang, S., One-pot synthesis of amphiphilic superparamagnetic FePt nanoparticles and in vitro magnetic resonance imaging. F.; Chaudret, B., Magnetic hyperthermia in single-domain monodisperse FeCo nanoparticles: Evidence for Stoner–Wohlfarth behavior and large losses.
Zhu, C.; Guo, S.; Fang, Y.; Dong, S., Reducing sugars: new functional molecules for the green synthesis of graphene nanosheets.
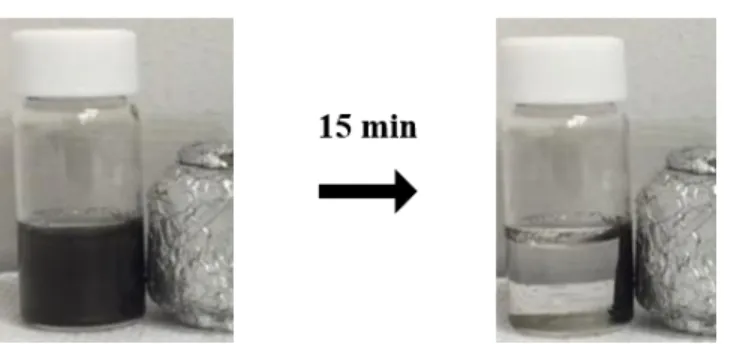