The influence of the target physical (thermodynamic and electrical) parameters on the plasma dynamics has been studied. A significant decrease in ablation efficiency (estimated as a function of ablated crater depth) with the increase in melting point was reported in [94].
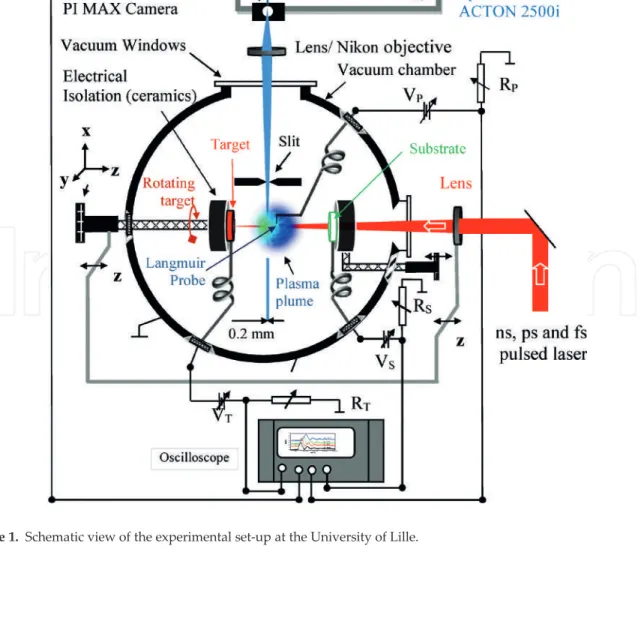
Theoretical investigations
An increase in the concentration of weak bonds results in an increase in the thermal and electrical conductivity of the glass. The spatio-temporal evolution of the variables describing the plasma agrees with other theoretical models [102, 103].
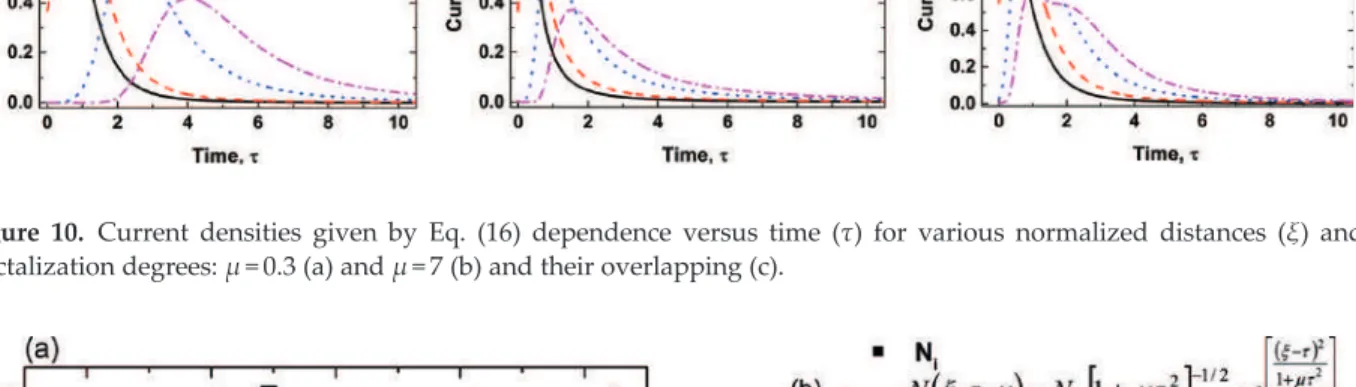
Conclusions
Dynamics of plume propagation and splitting during pulsed laser ablation of Si into He and Ar. Laser ablation of (GeSe2)100 x(Sb2Se3)x chalcogenide glasses: Influence of target composition on plasma plume dynamics.
Nanosecond Laser Ablation: Mathematical Models, Computational Algorithms, Modeling
Mazhukin
Introduction
The biggest differences in the physical mechanisms of laser metal removal are observed between short (ns) and ultrashort (ps, fs) pulsed modes [18, 19]. Laser ablation in the nanosecond range is a more complex phenomenon that involves many interrelated processes both during the action and after the end of the laser pulse. Such processes include heating of the laser target, heterogeneous and homogeneous phase transformations occurring in the vaporized matter, formation and expansion of the plasma plume, heat transfer, transfer of laser radiation and internal plasma, generation and propagation of shock waves and contact boundaries in the vaporized matter and the surrounding gas environment.
In these cases, the long-lived processes of the laser-plasma plume and the associated generation and propagation of interacting shock wave fronts and contact boundaries in the vapor-gas environment play an important role in the overall picture of laser ablation [27]. Each simulation begins with a choice of mathematical model, the construction and development of which is given great importance in computer experiments. The main shortcomings of the continuous approach are manifested in the lack of the possibility of direct investigation of elementary processes in materials and the limited possibilities of mathematical description of homogeneous mechanisms of phase transitions of the first type and calculation of thermophysical, thermodynamic, optical and other characteristics of matter in a wide range of parameters.
Primary attention is paid to modeling the dynamics of phase explosion of liquid phase of aluminum and the expansion of its fragments in the air, since explosive boiling is considered to be one of the most effective thermal mechanisms in ns laser ablation of materials. To obtain detailed information on interaction between heterogeneous and homogeneous mechanisms and data on laser plume morphology, simulation of laser heating, melting, surface evaporation and development of plume in the vapor-gas medium is carried out within the framework of a new hydrodynamic model with temperature dependence of material properties of the target and explicit boundary tracking of front, shock and shock.
Hydrodynamic model
The non-equilibrium of the KL is determined by the flow of matter through the phase boundary. In the heterogeneous evaporation model, a special role is played by parameter M(M = uυ/usoundis Mach number) on the outside of KL, which determines the degree of non-equilibrium of the phase transition. In the subsonic vaporization regime, when M<1, the behavior of the interface depends on gas dynamic perturbations in the vaporized material flow.
The values of the function F(T,M) depend weakly on parameter T and much more strongly on parameter M. Thus, the description of the kinetics of heterogeneous evaporation is performed by two dependencies on one parameter used as boundary conditions (for a known parameter M), and subsonic surface condensation is described by only one dependence on two parameters (M,T). The description of the processes in the expanding cavity and in the liquid layer near the surface separated from the main target was carried out using.
Also, the absorption and reflection of the intensity of the laser radiation in the formed layer has been taken into account. Subscripts 0 and 1 indicate the values of the quantities on the background side and the shock wave, respectively.
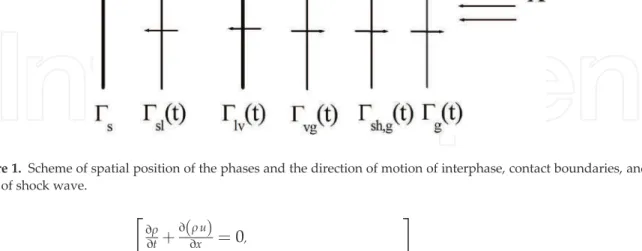
Temperature dependences of aluminum properties
The method is based on the idea of switching to an arbitrary non-stationary coordinate system that allows calculations with an arbitrary number of discontinuous solutions, such as shock waves, phase and temperature propagation fronts, contact boundaries and fragmented fragments.
Results discussion
In the initial phase, the time evolution of the processes at the surface of the target, in the target and in the gas medium near the target, such as the appearance of phase fronts (melting, vaporization), contact boundary and shock wave and associated with the formation of new phase media (liquid, vapor) at the leading edge of the laser pulse. When the interior of the target is heated, the melting front Гsl(t) runs from its surface and forms a new region of liquid phase. A stream of the vaporized substance was formed at the surface of the melt, pushing out air and creating another new phase - steam.
Therefore, when the target is strongly heated near the critical point (Tcr= 7600 K, ρcr= 0.47 g cm 3, pcr= 1.42 kbar), the thermophysical and optical properties of the liquid phase suddenly change. Thus, the results of simulations obtained using different methods indicate the presence of temperature inhomogeneity in the metal target (Al), which is caused by a decrease in the temperature of the irradiated surface due to heterogeneous evaporation. The first boiling occurs at the moment t = +1.5 ns, when the maximum of the temperature profile reaches the temperature of the limit overheating of the liquid phase, which is equal to Tℓ,max = 0.9Tcr = 6840 K.
Both the surface of the broken fragment and the surface of the target are subject to intense surface evaporation. As the fluence increases to F~ 10 J/cm2, the target heats up faster and the explosive boiling process starts moving towards the leading edge of the laser pulse.
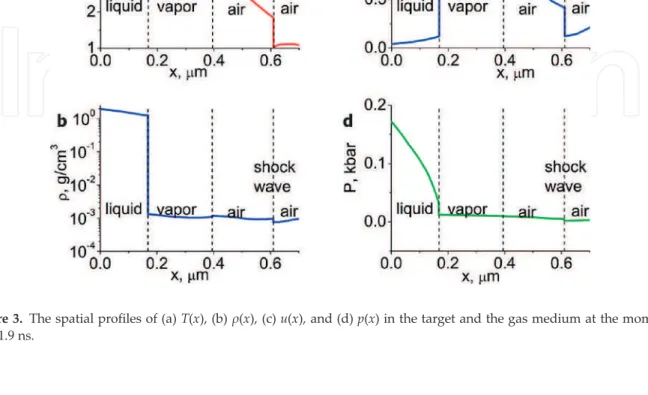
Conclusion
Experimental and computational study of complex shock wave dynamics in laser ablation plumes in argon atmosphere. A uniform model to determine the energy distribution between target and plasma in nanosecond laser ablation of silicon. Microscopic mechanisms of laser spallation and ablation of metal targets from large-scale molecular dynamics simulations.
Simulation of nanosecond pulsed laser ablation of copper samples: focus on laser-induced plasma radiation. 1D modeling of nanosecond laser ablation of copper samples in argon at P=1 atm with a wavelength of 532 nm. Evidence for phase explosion and large particle generation during high-power nanosecond laser ablation of silicon.
Modeling of plasma-driven evaporation and surface condensation of Al induced by 1.06 and 0.248 μm laser radiation.
Protected Laser Evaporation/Ablation and Deposition of Organic/Biological Materials: Thin Films Deposition
The ablation of organic/biological materials was initiated and promoted under "protection" and investigated at different stages after generation, during propagation in vacuum or different environments until the final deposition of expelled material on substrates of interest. Appropriate models were developed to describe the coupling of radiation to the organic/biological materials, bare or protected by a matrix, while additional techniques were used to obtain exhaustive information about the ablated substance in plasma plume, liquid and final solid state. The "protected" ablation and transfer of organics and biologicals can be achieved by multi-pulse laser irradiation.
It is now generally accepted that the main requirements for these choices are: laser fluence must have proper values, lower than in pulsed laser deposition (PLD); incident laser energy must be the majority absorbed by solvent molecules and not by organic molecules of the base material (0.5–10) wt.%; frozen solvent must be characterized by a high absorption at working laser wavelength; solvent must be chosen in such a way that the organic material offers a good solubility; solvent must have a high freezing point; and solvent must not produce chemical reaction under exposure to laser radiation. For illustration, we give in Figure 1 a general scheme of a MAPLE setup and the involved fundamental physicochemical processes in ablation of the frozen target. This makes the ablation process in the case of MAPLE substantially different from that in PLD.
Nevertheless, the selection of the perfect solvent is not easy and sometimes impossible due to the limits introduced by highly toxic characters of different solvents, available quantities and prices, and good mixing between a solvent and a solute, being the most important. Selected information is introduced on ablation and deposition of polysaccharides (tri-acetate-pullulan), enzyme (urease), proteins (fibro- and vitro-nectins) and biopolymers (papain, lysozyme, poly(lactic-co-glycolic acid).
2. “Generalized” MAPLE mechanism
Relevant examples
- Polysaccharides
- Proteins
- Enzymes
Part of the heat is transferred to the surrounding ice medium (c), the upper layer evaporates and the deeper layers freeze again (d) (reproduced with permission from [11]). The specific requirements of various applications can be met due to the possible adaptation of the properties of proteins [42]. The presence of the different side groups attached to the central carbon in the protein structure is an advantage for film production or even for its improvement (stability) because proteins can be subjected to chemical and/or mechanical modifications [42, 46].
Extracellular matrix (ECM) proteins present in all tissues and organs, fibronectin (FN), vitronectin (VN) and collagen I (Col1) have demonstrated their important role in wound healing processes as components [57]. We observed a weaker attachment and a round shape of the attached cells on the Ti control, while in the transferred samples the FNs were clearly expanded and the actin filaments were organized according to the aspect of the FN puncta [63]. FTIR micrographs of papain droplet (a) and thin films obtained by MAPLE (b) for 5 wt. % papain in distilled water and a laser fluence of 0.75 J/cm2 (reproduced with permission from [79]).
The presence of urea can be determined by electrochemical or optical methods on the basis of the ammonia formed [90]. The kinetic and enzymatic activity of the immobilized urease was evaluated using the Worthington assay method (Figure 9) [90].
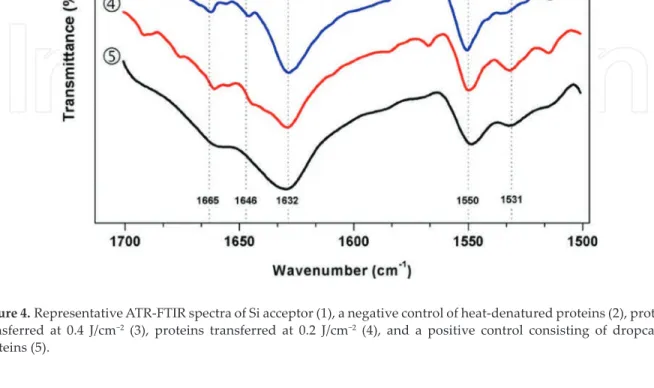
Conclusions
Acknowledgements
Author details
Comparative study on pulsed laser deposition and matrix-assisted pulsed laser evaporation of urease thin films. Dominique Helley and Sylvia Colliec-Jouault marine polysaccharides: a source of bioactive molecules for cell therapy and tissue engineering. 35] Cristescu R, Dorcioman G, Ristoscu C, Axente E, Grigorescu S, Moldavian A, Mihailescu IN, Kocourek T, Jelinek M, Albulescu M, Buruiana T, Mihaiescu D, Stamatin I, Chrisey DB.
Matrix-assisted pulsed laser evaporation processing of triacetate-pullulan polysaccharide thin films for drug delivery systems. 38] Jelinek M, Cristescu R, Axente E, Kocourek T, Dybal J, Remsa J, Plestil J, Mihaiescu D, Albulescu M, Buruiana T, Stamatin I, Mihailescu IN, Chrisey DB. Matrix-assisted pulsed laser evaporation of cinnamate-pullulan and tosylate-pullulan polysaccharide derivative thin films for pharmaceutical applications.
Fabrication of functional fibronectin patterns by nanosecond excimer laser direct writing for tissue engineering applications. Antimicrobial polycaprolactone/polyethylene glycol-embedded lysozyme coatings of Ti implants for osteoblast functional properties in tissue engineering.