Because the site is linked to electrodes, their interfaces determine the electrochemical reliability of the devices. Schematic illustration showing the direct printing-driven fabrication procedure of the pSEI-Li along with its chemical structure. Structural characterizations of the pSEI. a) Ionic conductivity and Li+ transfer number (tLi+)) of the pSEI film swollen with a liquid electrolyte (1 M LiPF6 in EC/DEC = 1/1 (v/v)) as a function of its composition ratio.
A plot of the mechanical modulus of pSEI (ETPTA/DADMA-TFSI = 30/70 (w/w)) as a function of penetration depth during the nanoindentation test. Water contact angle of (a) bare Li and (b) self-standing pSEI films as a function of DADMA-TFSI content. XPS (d) C1 and (e) F1s spectra of SEI formed at the QSIC-Li metal anode interface (versus liquid electrolyte).
The cycle performance was performed at a discharge current density of 1.0 C. c) Voltage profile and (d) cycle performance of the anodeless full cell configuration with QSIC and liquid electrolyte.
Introduction
- General background
- Electrolyte
- Liquid electrolytes
- Polymer electrolytes
- Inorganic single-ion conductors
- Organic single-ion conductors
- Composite electrolytes
- Ion transport phenomena
- In atomic scale
- In bulk scale
- References
Liquid state electrolytes have several advantages of highest ionic conductivity and excellent wettability. On the other hand, the sub-diffusive motion of the solvated ion cluster predominantly contributes to ion transport for oligomers (low molecular weight polymers). Compared to solid-state electrolytes, the main ion transport properties of GPEs are based on liquid bulk electrolytes, and ion conduction occurs in a mixed form of segmental transport and solvated ion transport only when polyanions are used.
To balance the mechanical and ionic properties, composite electrolytes of inorganic and polymer electrolytes are fascinating strategies.19 The beginning of composite electrolytes is to add non-conducting inorganic particles to polymer electrolytes, thereby increasing mechanical and thermal stability and inhibiting the formation of crystalline polymers, facilitating salt dissociation, accelerating ion transport at the limits of the inorganic polymer and providing processing diversity. Because of the practical limitations in accurately detecting electrochemical signals from a single conductive domain, ionic conductivity has been adopted as a measure for evaluating the conductivity of electrolytes. It should be noted that the convenience of measurement is based on the assumptions that the electrolyte has a uniform composition, distribution and orientation with uniform contact with the electrodes on an atomic scale, and its total ionic resistance is the average value of all unitary conductive domains located between the two electrodes.
The experimental error for an electrolyte in the liquid state is very low if it has no problems with the wettability and electron conduction of the electrode.
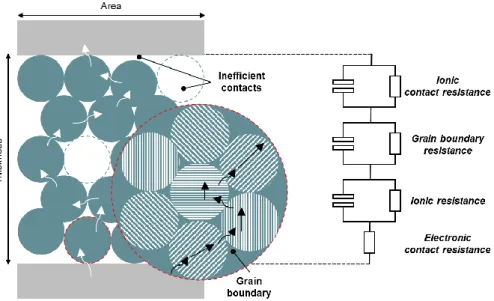
Design of organic single-ion conductor based on relaxation dynamics
Ion relaxation dynamics of G-quadruplex for single-ion conduction
- Introduction
- Experiment
- Results and discussion
- Conclusion
- References
Li + sliding behavior of LiGQ and its self-assembled crystalline structure were elucidated by in-depth experimental and theoretical studies. In addition, this diffraction pattern confirmed the hexagonal columnar order of LiGQ.26 The crystalline structure of LiGQ was further elucidated by TEM (Figure 2-4b). LiGQ prepared by non-solvent diffusion shows a hexagonal columnar order with the highest (001) peak intensity. b) TEM image of the LiGQ showing clear π-π stacking distance.
Based on the structural understanding of LiGQ described above, I investigated its feasibility as a single-ion conductor for potential use in Li batteries. The details of sample preparation and measurement conditions are described in "Cyclic voltammetry (CV)" in the experiment. By comparing the profiles of the blocking and non-blocking cells, the ionic transfer number for LiGQ (= 𝑖𝐿𝑖/(𝑖𝑒+ 𝑖𝐿𝑖))31 was estimated to be 0.95.
From potentiostatic polarization analysis, the Li+ transmission number of LiGQ was estimated to be 0.86, indicating the dominant contribution of Li+ to the ionic conductivity. This ionic conduction behavior of LiGQ was compared with previously reported monoionic conductors such as polyanions, inorganic conductors, covalent organic frameworks, and metal organic frameworks (Table 2-2). The synthesized NaGQ showed Hoogsteen hydrogen bonding and hexagonal ordering similar to that of LiGQ (Figure 2-7a and 2-7b).
The gradual decrease of the singlet 7Li peak during the cycling test (Figure 2-8b) confirms the Li+ conduction through the LiGQ.33 Furthermore, the Hoogsteen hydrogen bond and the hexagonal columnar arrangement of the LiGQ remained virtually unchanged (Figure 2-8c and 2-8d), which exhibits its structural durability during the. The unchanged Hoogsteen hydrogen bond and hexagonal arrangement of LiGQ showed structural durability of the LiGQ during the electrochemical test. e) SEM image of Li anode after the cycling test, showing a smooth surface without dendritic Li growth. This information shows that the Li-Nafion requires a thickness of 7.8 μm to synchronize its conductivity with that of the LiGQ.
This result confirms the viable role of the ion-dipole interaction in the directional Li+-sliding-driven fast ion transport of the LiGQ. The directional Li+ sliding of the LiGQ was enabled by its 1D central channels that enabled weak ion-dipole interaction and short and straight ionic paths. The hexagonally ordered columnar structure of the LiGQ was formed by the non-solvent diffusion-driven self-assembly of a guanine derivative with ion-responsive liquid crystalline moieties, ultimately producing the Li+-conducting 1D central channels.
Lee, H.; Yang, X.; McBreen, J.; Choi, L.; Okamoto, Y., The synthesis of a new family of anion receptors and the studies of their effect on the dissociation of ion pairs and the conductivity of lithium salts in non-aqueous solutions.
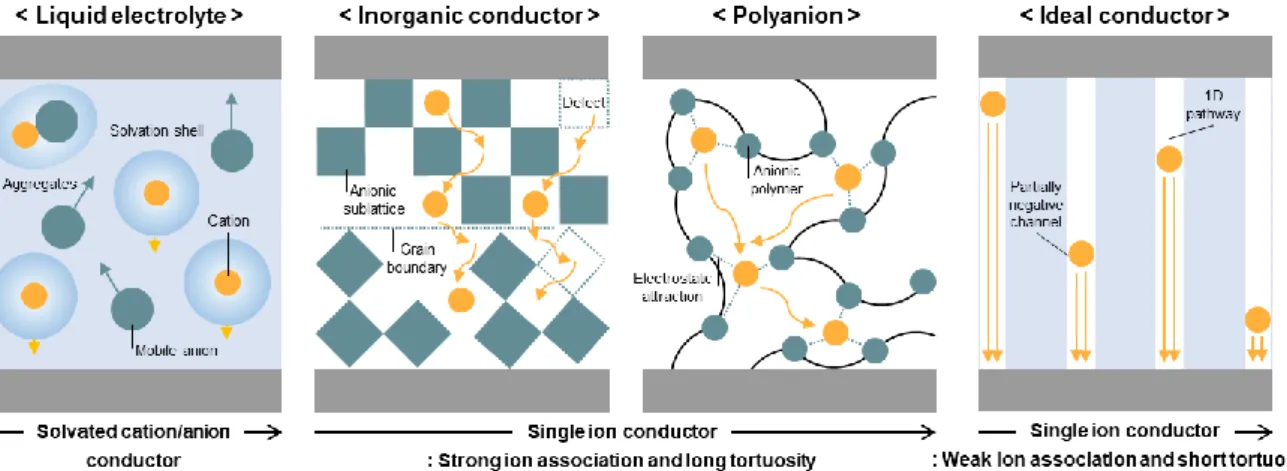
Design of organic single-ion conductor based on electrophoretic
Electrophoretic regulation of polycations for artificial solid-
- Introduction
- Experiment
- Results and discussion
- Conclusion
- References
The surface and cross-sectional morphologies of the pSEI-Li were characterized using a field emission scanning electron microscope (FE-SEM, S-4800 (Hitachi)) in conjunction with an energy dispersive X-ray spectrometer (EDS). The surface discoloration of the pSEI-Li was characterized by optical image analysis using digital camera under a controlled atmosphere. The fabrication procedure of the pSEI on Li metal, together with its chemical structure, is illustrated in Figure 3-1.
The pSEI paste (consisting of ETPTA and DADMA-TFSI, without any processing solvents) was printed directly on top of the Li metal thin foil and then polymerized by UV irradiation for 20 s, producing the monolithically integrated pSEI-Li. The ion conduction behavior of the pSEI was investigated as a function of its composition ratio (i.e., ETPTA/DADMA-TFSI). To better understand the high tLi + value, FT-IR analysis of pSEI was performed.
A noticeable shift of the characteristic peak assigned to the PF vibration of the PF6 anions was observed at pSEI (Figures 3–4c). pSEI-Cu showed uniform ionic topology with a low local resistance (~97 Ω cm2) after five Li plating/stripping cycles (Figures 3-5c), whereas the bare Cu showed an inhomogeneous ionic topology with random and high local resistance as a result of side reactions between the coated Li and liquid electrolytes (Figure 3-5d). The effect of pSEI on the antioxidation stability of Li metals was systematically investigated.
Meanwhile, the water contact angles of the pSEI films were measured with different composition ratios of ETPTA/DADMA-TFSI (Figure 3-6b). The water contact angle tended to decrease with increasing DADMA-TFSI content, indicating that the hydrophobicity of pSEI was predominantly derived from ETPTA. The surface tarnish test of pSEI-Li was performed as a function of exposure time under a humid environment.
We investigated the electrochemical activity of pSEI-Li after exposure to humid air (50% RH). Galvanostatic Li extraction of pSEI-Li against Cu foil was analyzed as a function of exposure time. This electrochemical viability of pSEI-Li combined with its structural stability (especially dimensional tolerance) is expected to benefit the volumetric energy density of Li metal full cells.
The pSEI (~1 μm) was introduced on the thin Li metal electrode (25 μm), which is highly desirable as a prerequisite for high energy density Li metal full cells.

Interpenetrated polycations for anode-free lithium storage
- Introduction
- Experiment
- Results and discussion
- Conclusion
- References
The structural evolution of the QSIC and the electrostatic interaction between the positively charged QSIC and the free anion were monitored using a Fourier transform infrared spectrometer (FT-IR, Alpha Platinum ATR (Bruker)). The surface and cross-sectional morphologies of QSICs were characterized using a field emission line electron microscope (FE-SEM, S-4800 (Hitachi)) in conjunction with an energy dispersive X-ray spectrometer (EDS). To investigate the pore size distribution, etched QSIC was prepared by solvent extraction (dimethyl carbonate followed by acetone) of QSIC.
QSIC showed characteristic Fourier transform infrared spectroscopy (FTIR) peaks of ETPTA (νO-C-O), cationic PU (νN-H14), and liquid electrolyte (νO=C-Li, νP-F) (Figure 3-10a). This structural characterization verifies that the QSIC has a homogeneous distribution of ETPTA, cationic PU and liquid electrolyte. The successful formation of semi-IPN structures was confirmed by examining the pore structures of QSIC after solvent extraction.
To highlight the fast Li+ transport behavior through QSIC-B(Ph)4, its Arrhenius plots of Li+ conductivity (estimated by ion conductivity × tLi+ ) were compared with those of liquid electrolyte with and without conventional polyethylene separator (Figure 3-11d and Figure 3-12). Due to the highly active state of Li cations, QSIC-B(Ph)4 showed the highest Li+ conductivity over a wide temperature range with the lowest activation energy compared to those of liquid electrolytes, even under a separator-free condition (black dots) in Figure 3-11d ). The QSIC-B(Ph)4 paired half-cell showed a very close open-circuit voltage with the liquid-electrolyte half-cell (QSIC-B(Ph)4 = 3.53 V, liquid electrolyte = 3.54 V vs. Li/ Li+), confirming that QSIC-B(Ph)4 could utilize all active particles.
The cyclic voltammogram of the cathode half-cell showed reversible Li intercalation and deintercalation via the QSIC-B(Ph)4. Moreover, the QSIC-B(Ph)4 effectively alleviates the cell polarization after the repeated current stimuli during the charge and discharge reaction in the galvanostatic intermittent titration technique analysis (Figure 3-15b), indicating the improvement in cathode kinetics due to its high Li+ conductivity. . Another notable advantage of the QSIC is its significantly low anion activity, even under the same conditions as using carbonate-based solvents (vs. liquid electrolyte).
The application of QSIC as a single-ion conducting electrolyte for lithium-ion battery and anode-less lithium storage was explored. Additionally, the QSIC anodeless cell showed greater capacity retention with cycling than the control electrolyte cell (Figure 3-19d). Driven by unique transport phenomena, QSIC enabled significant improvements in the electrochemical kinetics of both the high nickel oxide cathode and the Li metal anode.
Furthermore, QSIC was utilized as a solid-state electrolyte of an anode-free lithium metal battery as a proof-of-concept to explore its practical applicability.
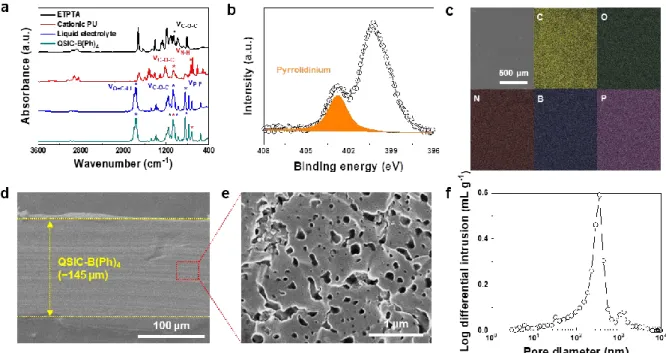